Science Highlights, June 8, 2016
Awards and Recognition
Capability Enhancement
New high resolution neutron capability developed at LANSCE
Turbulent Mixing Tunnel provides insight into buoyancy effects in mixing
High Performance Computing
First Open Science computing campaign completed on the Trinity-Haswell system
Program Leadership
Andersson, Maloy, McClellan, Stanek guide national efforts in nuclear energy
Babs Marrone chosen to participate in DOE Technologist in Residence Program
Los Alamos and Argonne national laboratories lead fuel cell catalyst development
Awards and Recognition
Doan Nguyen and co-authors win IEEE Van Duzer Prize
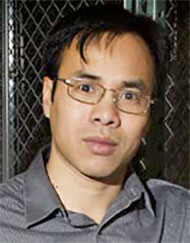
Doan Nguyen (Materials Physics Applications, Condensed Matter & Magnet Science, MPA-CMMS) and his co-authors will receive the 2014 IEEE Van Duzer Prize for their paper addressing AC losses, one of the most challenging technical problems for superconducting applications. Accurate prediction of AC loss in high temperature superconducting (HTS) wires/devices is needed to optimize the design of HTS systems. LANL provided a review on its advanced finite element model to accurately calculate AC loss and electromagnetic dynamics in a combined system of superconductors and ferromagnetic materials.
Reference: “Computation of Losses in HTS under the Action of Varying Magnetic Fields and Currents,” invited review paper in IEEE Transactions on Applied Superconductivity 24, 1, (2014); doi: 0.1109/TASC.2013.2259827. Authors: Francesco Grilli (Karlsruhe Institute of Technology), Enric Pardo and Fedor Gömöry (Slovak Academy of Sciences), Antti Stenvall (Tampere University of Technology), Doan Nguyen (MPA-CMMS), and Weijia Yuan (University of Bath).
The IEEE Council on Superconductivity sponsors the Van Duzer Prize to recognize the best contributed paper published in IEEE Transactions on Applied Superconductivity during each volume year. The award is named in honor of Professor Theodore Van Duzer, founding Editor-in-Chief of the journal and a member of the U.S. National Academy of Engineering. Judges evaluate papers on the expectation that the publication will be highly cited by future authors, technical excellence of the work, and completeness of the paper as an archival record of a finished body of research. The award will be given during the 2016 Applied Superconductivity Conference in Denver.
DOE’s High Temperature Superconductivity power applications projects, funded through LANL’s former Superconductivity Technology Center, sponsored the work. The Laboratory Directed Research and Development (LDRD) program funded a separate task to refine the model. The work supports the Lab’s Energy Security mission area and the Materials for the Future science pillar. Technical contact: Doan Nguyen
Glen Wurden named associate editor for Journal of Fusion Energy
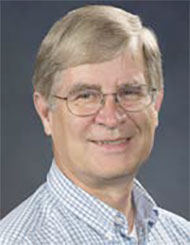
The Journal of Fusion Energy has chosen Glen Wurden (Plasma Physics, P-24) to serve as an associate editor. The journal publishes international research and review papers on the development of thermonuclear fusion as a power source, and provides a forum for discussion of broader policy and planning issues in energy fusion programs. Springer US publishes the journal six times a year. Wurden has published his work in the journal for the past 20 years.
Wurden received a PhD in astrophysical sciences/plasma physics from Princeton University. He joined the Laboratory in 1982 as a J. Robert Oppenheimer Postdoctoral Fellow. Wurden leads P- 24’s Magnetized Plasma team, which uses a wide range of plasmas and plasma diagnostic techniques to understand complex processes in hot fusion plasmas. He recently collaborated with the Massachusetts Institute of Technology and the Air Force Research Laboratory on tokamak and magnetized target fusion projects. Wurden also developed and installed cameras to image superhot plasmas at the new Wendelstein 7-X stellarator in Germany, where international researchers are testing an alternative approach to harnessing fusion energy. The American Physical Society has named him as a Fellow for his innovative approaches to plasma diagnostics applied to a wide variety of fusion confinement concepts, ranging from reversed field pinches to tokamaks to magneto-inertial fusion. Technical contact: Glen Wurden
Capability Enhancement
New high resolution neutron capability developed at LANSCE
A Laboratory team demonstrated greatly improved neutron energy resolution for nuclear science research at the Manuel Lujan Jr. Neutron Scattering Center at LANSCE. This improved energy resolution is necessary for LANL’s core mission in NNSA Defense Programs, which requires high-quality nuclear data in the neutron energy range from 10 keV to 1 MeV, for Subcritical Experiments, Nuclear Forensics, Stockpile Stewardship, and Radiochemical Diagnostics work.
LANSCE produces intense neutron beams. However, measurements in this middle-energy range are difficult due to low intensity and inadequate energy resolution. Currently, the Lujan Center neutron beam uses a target moderator system that is optimized for thermal neutrons and has a proton pulse width of 270 nsec (base width). To improve the performance in this middle-energy range and to meet the needs of Defense Program sponsors, two characteristics of the current neutron production system must be improved: 1) the epithermal neutron yield of the target and 2) the time resolution of the time-of-flight neutrons
The first parameter is being addressed by designing a new target that will increase the neutron production by a factor of 10 at 10 keV and more at higher energies. The wide proton pulse from the Proton Storage Ring (PSR) presents a major limitation to the neutron energy resolution. The width of the proton pulse must be reduced to achieve the required energy resolution.
Bob Macek (Accelerators and Electrodynamics, AOT-AE) proposed an innovative approach to achieving narrow pulses with less loss of intensity. In this method the short, high intensity beam pulses are obtained by a special radio frequency (RF) voltage program for the RF Buncher in the Proton Storage Ring. In a three-day collaborative experiment at the end of the 2015 run cycle, the team successfully demonstrated Macek’s approach and showed that the narrower beam pulse improved the neutron energy resolution. The investigators operated PSR in a “pulse-stacking” mode where several hundred 5-ns linac beam micropulses are injected on top of each other and then bunched to produce a short high-intensity pulse. During neutron scattering measurements made with the shorter pulses, the researchers observed neutron resonances that were not resolvable with the standard 270 ns proton pulse. The tests resulted in approximately a factor of 9 narrower pulse (30 ns) with only a factor of three loss of intensity.
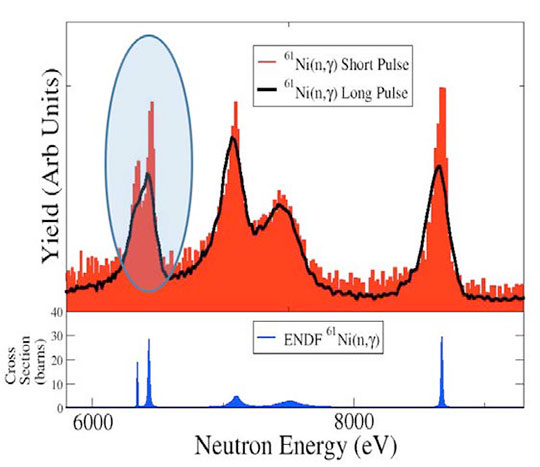
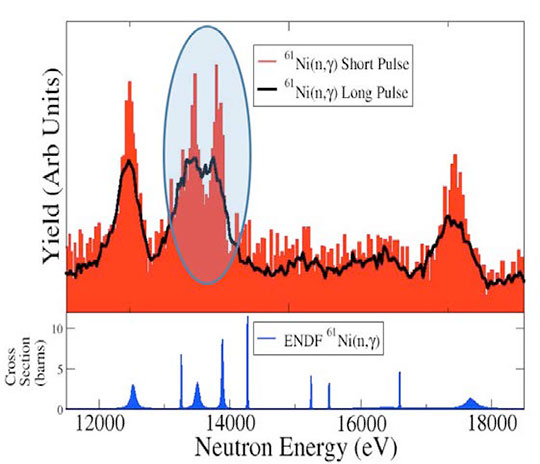
Figure 1. (Top): The 61Ni(n,γ) capture yield between 6 and 10 keV. The circled area shows the effect of the improved resolution. (Bottom): The 61Ni(n,γ) capture yield between 11 and 19 keV. The circled area shows the effect of the improved resolution.
The team used DANCE (Detector for Advanced Neutron Capture Experiments) with the standard 270 ns pulse and a narrow 30 ns wide pulse to acquire data on a neutron capture reaction with nickel-61 (61Ni). In this reaction, 61N captures a neutron, producing 62Ni and a gamma ray [61Ni(n,γ)62Ni]. Figure 1 (top) shows the capture yield measured with the wide pulse (270 nsec, black), the 30 nsec pulse (red) and the ENDF evaluations (blue) from 6 keV to 10 keV. With the wide pulse, the resonances at approximately 6.5 keV appear as a single broad peak (black line). With the narrow pulse, the peak is resolved into two resonances (red data) as predicted by the ENDF evaluation (blue). In Figure 1 (bottom) the broad structure between 13 and 14 keV seen with the wide proton pulse is resolved into two peaks as predicted by the ENDF evaluation.
The measurements have demonstrated a proof-of-principle way to improve the neutron energy resolution. The team plans a complete MCNP (Monte Carlo N-Particle) simulation of the neutron production process, exploration of the tradeoffs between pulse width and intensity, and doubling the repetition rate. This would bring short-pulse intensity to within 60% of the nominal operating intensity. Resolving resonances would allow new science and measurements for LANSCE.
The team included: A. Couture, S. A Wender, and J. L. Ullmann (LANSCE Weapons Physics, P- 27); R. J. Macek (Accelerators and Electrodynamics, AOT-AE); T. Spickermann, R. McCrady, and J. Kolski (Accelerator Operations, AOT-OPS); and M. Prokop (Radio Frequency Engineering (AOE-RFE). NNSA funded the work, which supports the Lab’s Nuclear Deterrence and Global Security mission areas and the Applied Nuclear Science and Engineering (ANSE) focus area of the Nuclear Particle Futures science pillar. Technical contact: Steve Wender
Turbulent Mixing Tunnel provides insight into buoyancy effects in mixing
The Turbulent Mixing Tunnel (TMT) is a new, open-circuit wind tunnel facility designed to study jet and shear flow conditions for NNSA. Its unique diagnostic suite and multi-fluid capability make it the premiere source for experimental data on variable density mixing in shear conditions. The TMT facility has capabilities for many experimental configurations, including studying transient flow conditions, multiple/colliding jets, and other flow geometries.
Researchers use TMT data to validate turbulence models for variable-density mixing conditions. Particle image velocimetry (PIV) and planar laser induced fluorescence measure the velocity and density fields simultaneously in two dimensions. Current experiments study the effects of changing the Atwood number of the jets with matched Reynolds numbers. (The Atwood number is a function of the density ratio of the jet to the background material; the Reynolds number is the ratio of inertial forces to viscous forces.) Jets provide a useful configuration for studying mixing because the jet does not mix with the surrounding air in a uniform way. Intermittent, large-scale structures characterize the turbulence. The turbulence that does not necessarily behave the way the models that have been implemented into numerical codes might predict.
Density-weighted turbulence quantities in variable-density jets reveal flow dynamics that are highly dependent upon initial conditions, with buoyancy effects that evolve in space and time. Both turbulence and mean quantities are sensitive to these effects. Turbulence quantities are particularly revealing of important turbulent jet behaviors.
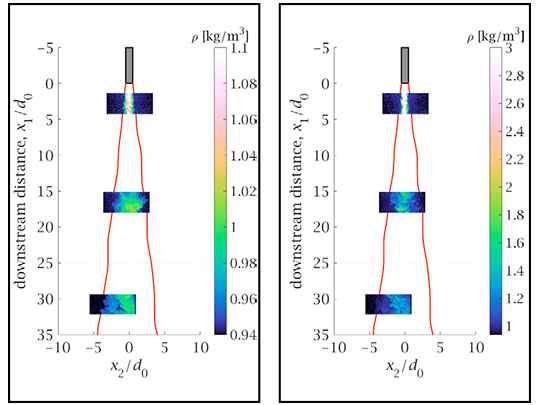
Figure 2. The measurement regions for air (left) and sulfur hexafluoride (SF6) (right) jets are shown to scale.
The team acquires 10,000 simultaneous density and velocity fields in each of these downstream locations. Figure 3 depicts an example of one such field. The spatial resolution and accuracy of particle image velocimetry allows resolution of the wide range of spatial scales that are present in the flow field. The team calculated mean quantities using all 10,000 images, and fluctuations by subtracting the mean field from each of the instantaneous fields.
Examination of the turbulent kinetic energy (TKE) clarifies the trends seen in the Reynolds stresses. This density-weighted quantity reveals how much total kinetic energy is in the density and velocity fluctuations of the flow field. TKE increases dramatically for the SF6 jet from the near field to the buoyancy-dominated region. Even at 30 diameters downstream, the TKE is still higher in the SF6 jet, even though it began with a lower TKE than the air jet. The team measures other important experimental quantities for the BHR (Besnard Harlow Rauenzahn) turbulence model, such as turbulent mass flux and density-specific volume covariance. Researchers compare the data with simulations. Normalization with the local value shows much higher dissipation rates in the air jet.
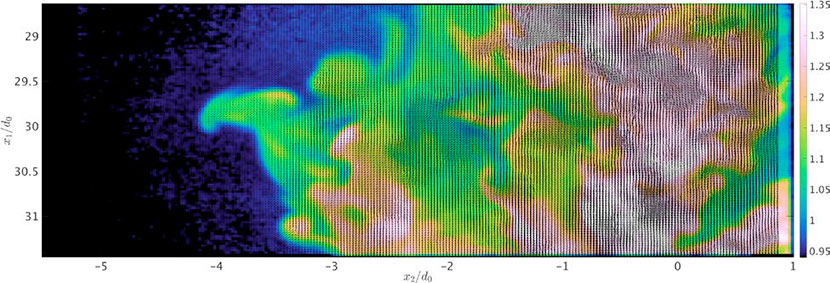
Figure 3. Density and velocity fields. The color scale is density in kg/m3. The field also constrains velocity vectors. There are vectors even in the dark regions.
NNSA funded the work at the TMT. The capability supports the Laboratory’s Nuclear Deterrence mission area and the Nuclear and Particle Futures science pillar by providing experimental fluid dynamics and turbulence measurements to validate models. The team includes John Charonko and Kathy Prestridge (Neutron Science and Technology, P-23). Technical contact: Kathy Prestridge
Chemistry
Testing hiRX instrument for improved plutonium assay at Savannah River National Lab
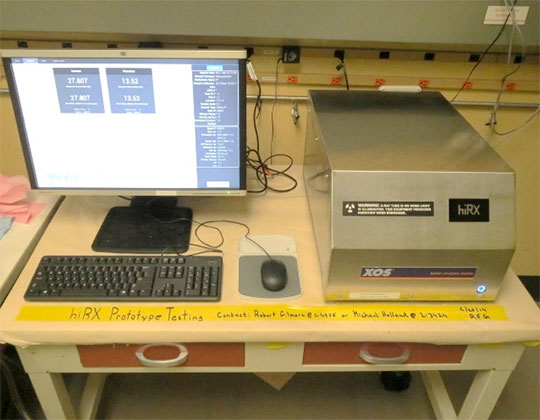
Photo. hiRX prototype instrument at SRNL.
Testing hiRX instrument for improved plutonium assay at Savannah River National Lab Safeguards require accurate, precise, and timely determination of plutonium (Pu) concentrations in nuclear fuel reprocessing plant systems. Current methods are either indirect, utilize significant sample preparation methodologies, or require long counting times. The considerable measurement time required and uncertainty contributed by use of corrections in determining Pu value may enable undetected diversion events to occur. LANL researchers developed high resolution X-ray (hiRX), a new analytical instrument that uses novel doubly curved crystal (DCC) optics to achieve high sensitivity and selectivity for actinide characterization. The hiRX offers a rapid, direct method for quantitative analysis of Pu concentration in high radiation reprocessing streams.
Chemists tested a prototype hiRX instrument in Savannah River National Laboratory’s (SRNL) F-area as part of the H-Canyon test bed program. The benchtop instrument consists of a compact X-ray tube, excitation DCC, collection DCCs for Pu and uranium (U), and a silicon drift detector. A door to access the sample chamber and instrument control via standalone PC make the hiRX easy to operate. The photos depict the simple procedure to prepare samples for analysis. An operator pipets seven microliters of sample solution into disposable polycarbonate microcells and mounts them in the instrument for measurement. Any liquid sample can be measured directly without additional sample preparation.
The team tested hiRX performance with Pu and U process samples taken from Savannah River Site’s (SRS) H-Canyon and HB-Line nuclear facilities. Chemists calibrated the instrument with reference materials that SRNL had prepared. Because U can be present at up to 100 times the Pu content in process samples, the researchers completed one-time calibrations for Pu only and for Pu in U-rich matrices. The hiRX measured the samples for 100 seconds. Results based on Fundamental Parameters analyses of dissolved spent fuel and process solutions showed biases of ≤5% for Pu and U versus routine accountability methods, with between-microcell repeatability of <5% relative standard deviation. Calculated Pu detection limits are on the order of 3x10-5 to 2x10-3 g/L dependent on matrix U content.
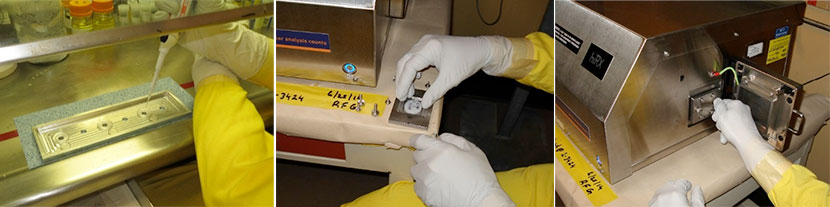
Photos. Steps in the hiRX sample preparation process including pipetting 7 microliter, placing the capped microcell in holder, and mounting the cell in the sample chamber.
As a result of the successful testing, SRNL scientists have identified several applications for hiRX in the F-area Analytical Laboratory in support of SRS nuclear material processing, material stabilization, and waste handling missions. These include Pu and U measurements for process control, material control and accountability, material characterization, waste characterization, and product specification. Major advantages of hiRX include reduced waste, enhanced safety, and rapid results without significant sample preparation. The hiRX technology also offers flexibility in sample type and instrument configuration, such as online and person-portable versions.
Reference: “hiRX Performance Testing at SRNL using Spent Nuclear Fuel,” Los Alamos National Laboratory Report LA-UR-16-20357 Version 2. Authors include: Kathryn G. McIntosh (Chemical Diagnostics and Engineering, C-CDE), George J. Havrilla (Chemistry Division, CDO), Robert F. Gilmore, Jr. and Michael K. Holland (Savannah River National Laboratory).
The DOE International Safeguards program, Next Generation Safeguards Initiative (NA-241) funded the work, which supports the Lab’s Global Security and Nuclear Deterrence mission areas and the Science of Signatures science pillar through development of actinide analytical methods. Technical contact: George Havrilla
High Performance Computing
First Open Science computing campaign completed on the Trinity-Haswell system
Trinity is a major high performance computer acquisition funded by the NNSA’s Advanced Simulation and Computing (ASC) Program, and developed by the New Mexico Alliance for Computing at Extreme Scales (ACES), a collaboration between Los Alamos and Sandia national laboratories. Trinity is being built by Cray, Inc., and will be installed in two phases. Phase 1 is based on Intel Haswell processors, and Phase 2 is based on Intel Xeon Phi Knight’s Landing (KNL) processors. Trinity will provide increased computational capability for the NNSA Nuclear Security Enterprise in support of ever-demanding workloads, e.g., increasing geometric and physics fidelities while maintaining expectations for total time to solution. The capabilities of Trinity are required for supporting the NNSA Stockpile Stewardship program’s certification and assessments to ensure that the nation’s nuclear stockpile is safe, reliable, and secure. As part of its Trinity system initial stabilization activities, the Lab made large parts of the system available to a select group of users for open science.
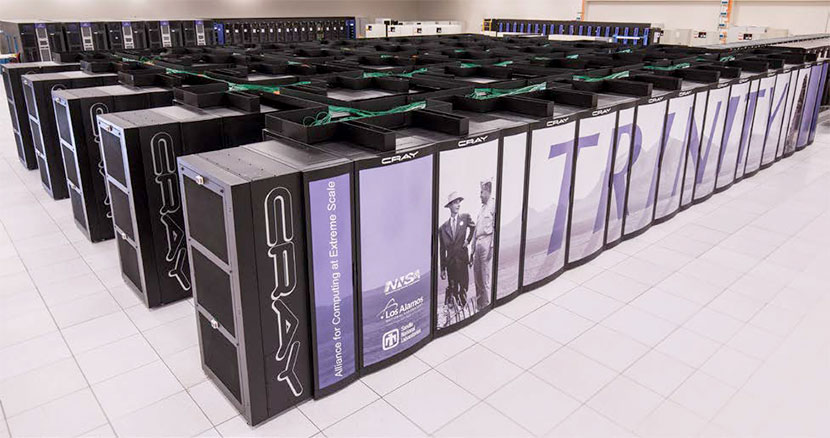
Photo. Trinity-Haswell system.
Researchers completed a two and a half month Open Science computing campaign after the Phase-1 system was installed and integrated at LANL. The Open Science campaign enabled select teams of scientists to investigate problems previously not possible without Trinity’s massive processing and memory capabilities. These problems improve the scientific knowledge and capability of the Laboratory, which underpin the Nuclear Deterrence mission area. The campaign period is also used to stabilize and validate the new hardware and software by running applications at large scale, consuming most or all of the processors of the machine, before the machine shifts to a production mode. End users running large scientific applications during the open science period stress the system in new and unique ways, enabling computer scientists to identify and resolve important configuration and stability problems. This accelerates the effort to create a stable and productive capability for production users. During the Open Science campaign, the entire Trinity resource was devoted to eight application teams from Los Alamos, Sandia, and Livermore national laboratories. Researchers investigated important problems in plasma and materials physics, next generation energy creation technologies such as fusion and wind turbine blades, genomic processes, and nuclear terrorism events. Los Alamos projects and principal investigators included:
- Helium Bubble Growth in Tungsten under Fusion First-Wall Conditions - Arthur Voter (Physics and Chemistry of Materials, T-1)
- Kinetic Plasma Modeling Using VPIC for Particle Accelerations and Plasma Mix Studies - Brian Albright (XTD-Primary Physics, XTD-PRI)
- Direct Numerical Simulations of Magnetic Rayleigh-Taylor Instability - Daniel Livescu (Computational Physics and Methods, CCS-2)
- Task-Based Adaptive Physics Refinement for Materials Simulation: Petascale Demonstration and Evaluation of a Burst Buffer Database Approach – Tim Germann (Physics and Chemistry of Materials, T-1)
Some of the Open Science projects were selected and funded as part of the Laboratory Directed Research and Development (LDRD) review process. The LDRD funding focused on specific scientific research relating to adapting software to the new Intel Xeon Phi (Knights Landing) processors or innovative use of new Burst Buffer hardware and software unique to Trinity. Research results will be published in major scientific journals over the next year. Some of these projects will run related problems during the planned Trinity Phase 2 Open Science period at the end of 2016.
Trinity is the first Advanced Technology (AT) system for the NNSA Advanced Simulation and Computing (ASC) Program. It will implement the new computing strategy requiring all AT systems to service NNSA mission workload while preparing the ASC applications for transition onto future advanced architectures. Los Alamos, Lawrence Livermore, and Sandia national laboratories will use Trinity. Trinity supports the Laboratory’s Nuclear Deterrence mission area and the Information, Science, and Technology science pillar through advanced computing capabilities. The Open Science campaign supports LANL’s Global Security, Nuclear Deterrence, and Energy science mission areas. Technical contacts: Bob Tomlinson (Institutional Computing, Trinity Open Science), Jim Lujan (ASC Platforms), and Bill Priedhorsky (LDRD).Physics
Examining the role of amyloids in type II diabetes
A collaboration between Los Alamos, Yale University, and Worcester Polytechnic Institute published research in Langmuir that sheds light on pathological properties of amyloids identified in type II diabetes. Amyloids are unwanted aggregates of proteins in our bodies. Frequently they form fibers whose presence is correlated with the pathology for many diseases, including Alzheimer’s, Parkinson’s, and type II diabetes.
Type II diabetes is a progressive disorder that has no known cause or cure. It affects approximately 28 million Americans and is the seventh leading cause of death in United States. The inability to maintain proper blood glucose levels defines type II diabetes. At the cellular level, loss of sensitivity to insulin, a hormone regulating the metabolism of carbohydrates, in the peripheral tissues results in increased insulin production by pancreatic β-cells. Secretion of insulin is also associated with increased production of islet amyloid polypeptide (IAPP), a short 37 amino acid peptide chain. The increase in amyloid secretion and aggregation into fibrils destroys the β-cells, subsequently leading to insulin deficiency. The molecular mechanism by which the cell death occurs is not yet understood, but evidence points towards interactions of IAPP oligomers with cellular membranes leading to breakdown of their integrity. Understanding the structural details of the lipid membrane and its interaction with IAPP could help identify the mechanisms of membrane disruption and enable the development of strategies to fight type II diabetes and other physicochemically similar diseases.
The research team used neutron surface scattering and fluorescence microscopy methods at Los Alamos Neutron Science Center’s Lujan Center to study interactions of IAPP with formulations of supported lipid model membranes. Neutron scattering delivers high spatial resolution, penetrability, and good scattering contrast for biological materials. This unique tool enables studies of supported lipid bilayer membranes and their interactions with organic molecules. The measurements could provide insight into the mechanism of toxicity of the amyloid and preamyloid toxins on the structure and integrity of the lipid bilayer.
The investigators examined interactions of human and rat islet amyloid polypeptides with the model membranes. Human IAPP (hIAPP) is toxic and prone to form amyloids, whereas the rat IAPP has reduced toxicity and does not form fibrils under physiological conditions. Both peptides are the same length, and their sequences differ by only a few amino acids. The hIAPP assumes a largely β conformation and oligomerizes, and the more soluble rat IAPP has a random conformation. These phenomena may result from the difference between serine (human) and proline (rat) at position 28 in the peptide sequence.
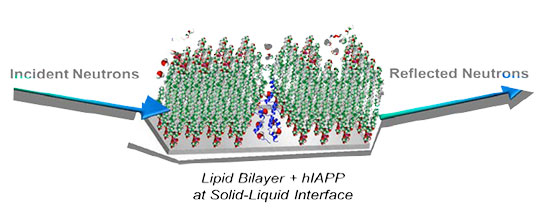
Figure 4. Schematic of human IAPP interaction with a DOPG lipid bilayer and corresponding calculated scattering length density (SLD) profiles. DOPG is a negatively charged phospholipid molecule with one unsaturation per chain. The bilayer structure and stability of anionic unsaturated DOPG are sensitive to amyloid protein interaction, and the hIAPP partially solubilized the bilayer under these conditions.
The research showed that human IAPP had little or no effect on most of the lipid membrane formulations studied. However, the membrane structure and stability of negatively charged and unsaturated lipids was extremely sensitive to interaction with hIAPP, and amyloid aggregates partly destroyed the membrane. Negatively charged lipids normally localize in the inner leaflet of the cell membrane. When the bilayer is damaged, the lipids can be present in the outer leaflet and interact with biological molecules like hIAPP. In contrast, rodent IAPP had no perturbing effects on any of the membrane formulations.
The authors suggested that following factors might contribute to the strong interaction of hIAPP with the DOPG bilayer: polypeptide fibril or protofibril formation, high local concentration (clustering) of anionic unsaturated lipids, relaxed molecular packing due to unsaturated tails, and macroscopic defects in the structure of the membrane. Because the rat IAPP did not form fiber structures in solution nor perturb the structures of the model membranes, the team speculates that the observed effect of hIAPP on the model lipid membrane might be connected with its ability to form aggregates. The team concluded that hIAAP disintegrates the DOPG lipid bilayer via folding and removing large patches of the membrane rather than by linking single (or limited numbers) of lipid molecules.
Reference: “Influence of the Human and Rat Islet Amyloid Polypeptides on Structure of Phospholipid Bilayers: Neutron Reflectometry and Fluorescence Microscopy Studies,” Langmuir 32, 4382 (2016): doi: 10.1021/acs.langmuir.6b00825. Authors: Ann Junghans (Engineering Materials, MST-7), Erik B. Watkins Materials Synthesis and Integrated Devices, MPA-11), Jaroslaw Majewski (Center for Integrated Nanotechnologies, MPA-CINT), Andrew Miranker (Yale University), and Izabela Stroe (Worchester Polytechnic Institute).
This research used resources of the Manuel Lujan Jr. Neutron Scattering Center, which was a DOE Office of Science User Facility at that time the data used in this publication were collected. The research supports LANL’s Global Security mission area and Materials for the Future and Science of Signatures science pillars through the study of complex and living systems to understand the molecular basis of disease. Technical contacts: Ann Junghans, Erik Watkins, and Jarek Majewski
Program Leadership
Andersson, Maloy, McClellan, Stanek guide national efforts in nuclear energy
The DOE Office of Nuclear Energy (NE) has chosen Chris Stanek, David Andersson, Stuart Maloy, and Ken McClellan (Materials Science in Radiation and Dynamics Extremes, MST-8) for leadership roles. These scientific leaders work with collaborators worldwide to develop future nuclear reactor designs and fuel cycle options.
David Andersson has joined the Consortium for the Advanced Simulation of Light Water Reactors (CASL) leadership team as a Deputy Focus Area Lead for Fuels, Materials and Chemistry. He collaborates with Focus Area Lead Brian Wirth (University of Tennessee) and Deputy Focus Area Lead Richard Williamson (Idaho National Laboratory) to guide the development of material models and simulation capabilities enabling CASL to address core challenge problems, including pellet-cladding-interaction and deposition of corrosion products on cladding. Los Alamos is a founding partner of CASL, which was established in 2010 to develop modeling and simulation technology for a virtual version of existing operating nuclear reactors.
Stuart A. Maloy is Reactor Materials Technical Lead for the Nuclear Energy Enabling Technologies (NEET) program. His position is part of the Reactor Materials crosscut effort supporting the development of revolutionary materials and providing broad-based, modern materials science benefitting DOE-NE objectives. This is accomplished through innovative materials development, promoting the use of modern materials science and establishing new, shared research partnerships. Maloy provides management support and fosters integration across DOE-NE programs, other offices, and international interests.
Ken McClellan is the Advanced Light Water (LWR) Reactor Fuels Thrust Area Lead for the Advanced Fuels Campaign under DOE-NE’s Fuel Cycle R&D program. He is responsible for overall coordination and integration of the various light water reactor fuel activities at the national laboratories. A primary focus is development of fuels with enhanced accident tolerance for application in commercial power reactors. In addition to the national laboratory activities, he also supports integration of university and industry-led LWR fuel technology development projects as well as international collaborations.
Chris Stanek was named the National Technical Director for the Nuclear Energy Advanced Modeling and Simulation (NEAMS) program, which develops, applies, deploys, and supports a predictive modeling and simulation toolkit for the design and analysis of current and future nuclear energy systems. The toolkit uses computing architectures from laptops to leadership class facilities. Los Alamos primarily contributes to the advanced nuclear fuel development by developing multiscale models of fuel performance.
Nuclear power plants generate more than 50% of the world’s clean energy. DOE’s Office of Nuclear Energy’s mission is “to advance nuclear power as a resource capable of meeting the Nation’s energy, environmental, and national security needs by resolving technical, cost, safety, proliferation resistance, and security barriers through research, development, and demonstration as appropriate.” Technical contact: David Teter
Babs Marrone chosen to participate in DOE Technologist in Residence Program
Biologist and Biofuels Program Manager Babs Marrone (Bioenergy and Biome Sciences, B-11) has been chosen as one of the first participants in the DOE Energy Efficiency and Renewable Energy’s Technologist-in-Residence (TIR) program. TIR is an innovative pilot program to build long-term relationships between manufacturing companies and the national laboratories in order to promote collaboration on innovative R&D. The program pairs senior technical staff from manufacturing companies with leading technologists from the labs, such as Marrone. This allows Lab and industry researchers to work together to more deeply understand the true nature of industry’s most important problems and the Lab capabilities that can best be brought to bear to solve them.
Through the TIR program, Marrone is working with James McCall, Global Product Supply Sustainability Leader at Procter and Gamble (P&G). McCall had previously collaborated with LANL and other national labs. He seeks to expand the partnership by working collaboratively to improve sustainable manufacturing and distribution processes— specifically related to buildings, transportation, water, and waste.
P&G has set ambitious goals for reducing the environmental footprint of its 28 manufacturing facilities in the U.S. and 100 facilities worldwide. The company has committed to reduce facility energy use by 20 percent per production unit, reduce greenhouse gas emissions by 30 percent, and power its plants by 30 percent renewable energy by 2020.
Marrone first visited P&G to meet the Technology and Chemicals teams and better understand their needs. McCall and Marrone are now working to identify laboratory staff, expertise, and resources that can be leveraged to tackle P&G’s sustainability challenges. Formal relationships and agreements will then be established between P&G and the applicable lab, aiming for a streamlined two- to three-year implementation timeline that is much faster than the traditional R&D process. More information: http://energy.gov/eere/cemi/technologist-residence-program
The Program advances several of the Administration’s priorities, including enhancing U.S. manufacturing competitiveness and the commercial impact of the DOE’s national laboratories. The work supports the Lab’s Energy Security mission area through the development of methods to reduce the carbon footprint and energy consumption of manufacturing processes. Technical contact: Babs Marrone
Los Alamos and Argonne national laboratories lead fuel cell catalyst development
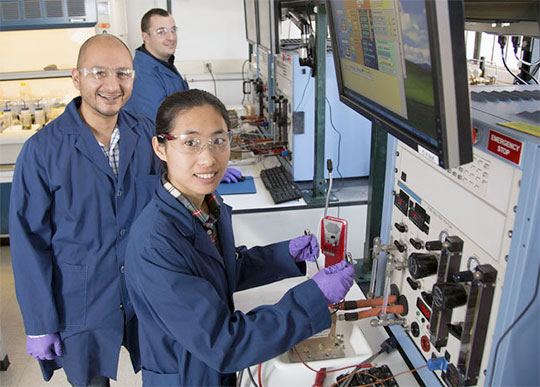
Photo. Inside the Los Alamos National Laboratory fuel cell test lab, graduate students and a post-doctoral researcher work on ElectroCat technology–from rear, Joseph Dumont, Ulises Martinez, and Ling Lin.
Researchers at Los Alamos and Argonne national laboratories have teamed to support a DOE initiative through the creation of the Electrocatalysis Consortium (ElectroCat), a collaboration devoted to finding an effective but cheaper alternative to platinum and platinum group metals in hydrogen fuel cells. ElectroCat aims to increase U.S. competitiveness in manufacturing fuel cell electric vehicles and other fuel cell energy conversion devices by addressing the primary challenges to the widespread implementation of this technology. The precious metal electrocatalysts that are the current standard in fuel cell systems are expensive and restrict the ability to develop fuel cells that are cost-competitive with traditional hydrocarbon-based power sources. Catalyst design and cost represent the most pressing material barrier related to fuel cell deployment and cost-competitiveness with traditional hydrocarbon-based power sources. ElectroCat aims to find new ways to replace rare and costly platinum group metals in fuel cell cathodes with more accessible and inexpensive substitutes–such as materials based on the earth-abundant and less expensive metals iron and cobalt.
Accelerating the development of effective platinum group metal-free (PGM-free) electrocatalysts requires a systematic approach that employs high-throughput, combinatorial, and computational methods to direct discovery and development. ElectroCat aims to streamline access to unique and powerful tools across the national laboratory network, to help develop missing strategic capabilities, and to curate a public database of information that will serve to enable the incorporation of PGM-free catalysts and electrodes into next-generation fuel cells.
Argonne National Laboratory and Los Alamos National Laboratory co-lead ElectroCat. Piotr Zelenay (Materials Synthesis and Integrated Devices, MPA-11) directs the Los Alamos component of the consortium. By combining the expertise and capabilities at Los Alamos and Argonne, in partnership with the private sector and universities, researchers expect to accelerate the development and implementation of platinum group metal-free catalysts in fuel cells.
ElectroCat is one of four consortia that make up DOE’s Energy Materials Network (EMN). The EMN will facilitate industry access to the unique scientific and technical resources available at the national laboratories, enabling manufacturers to bring advanced materials to market more quickly. Each EMN consortium will bring together national labs, industry, and academia to focus on specific classes of materials aligned with industry’s most pressing challenges related to materials for clean energy technologies.
The partnership between Argonne and Los Alamos that forms the core of ElectroCat involves the study, creation, and implementation of possible alternatives to platinum-based electrodes through material-development efforts headed by Los Alamos and accelerated by the high-throughput, combinatorial, characterization, and electrode performance modeling capabilities at Argonne, plus application of high-performance supercomputing to model new catalyst structures at LANL.
LANL has more than 35 years of experience in the design, development, and integration of fuel cell electrodes and membrane-electrode assemblies. In developing new materials to be explored for ElectroCat, researchers at Los Alamos will bring to bear 15 years of experience in nonprecious metal catalyst design, synthesis, characterization and testing. The capabilities include catalyst and catalyst support synthesis and synthesis of “model” catalysts via the controlled introduction of heteroatom and metal dopants using neutral atom beam technology (ENABLE) and other techniques. Characterization at the Los Alamos Neutron Science Center and nano x-ray computed tomography provide materials characterization capabilities. Multiscale modeling techniques leverage world-class computing facilities to design catalysts with optimal activity, selectivity and durability. These capabilities enable the close integration of modeling and experiment.
Once the potential replacement candidate materials are identified, consortium scientists will examine materials using a number of different methods, including x-ray imaging and spectroscopy techniques at Argonne. Argonne researchers can also investigate samples in a number of different environments that replicate how they would function in real-world scenarios.
The Fuel Cell Technologies Office in the DOE Office of Energy Efficiency and Renewable Energy funds ElectroCat. The research supports LANL’s Energy Security mission area and Materials for the Future science pillar through the development of materials for clean energy. Technical contact: Piotr Zelenay
Theoretical
Spontaneous popping of particles out of a vacuum
The journal Physical Review Letters reports an interesting phenomenon of magnetic materials. The magnetization in magnetic materials can be regarded as a little arrow pointing in certain direction in space. In ferromagnets, the arrows align in the same direction. Scientists had noted in the 1970s and later that the arrows could self-organize into a peculiar localized texture due to the underlying microscopic interactions. In the language of field theory, the uniform ferromagnetic state can be regarded as a vacuum state and the localized magnetization texture is a particle-like excitation. Generally creating a “particle” in “vacuum” requires overcoming an energy barrier and costs energy. Therefore the particle is a metastable state. In the new publication, Los Alamos researchers and collaborators found that in certain magnets, the “particle” has lower energy than the “vacuum” once an impurity is introduced into the system, resulting in the spontaneous nucleation of “particles” out of a “vacuum”.
One type of magnetization texture called skyrmion, named after former Los Alamos scientist Tony Skyrme, attracted considerable attention soon after its experimental observation in magnets without inversion symmetry. As shown in Figure 5, a skyrmion is a swirling spin texture with a unique topology. A skyrmion is a metastable excitation in the ferromagnetic state. It has a finite lifetime and disappears in the presence of fluctuations.
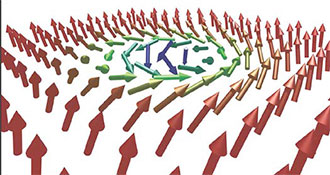
Figure 5. Schematic view of a skyrmion magnetization texture. Arrows denote the magnetization direction.
The team investigated magnets with inversion symmetry and with competing interaction between magnetic ions. An example is the rare earth magnets. The ground state is no longer the ferromagnetic state due to competing interaction. Instead, the magnetization arrows rotate in space and stabilize a magnetic spiral state. Under a magnetic field, all the magnetization arrows are polarized by the external field. This forces the system to be ferromagnetic. The researchers what would happen if a nonmagnetic impurity, which could be produced by removing one magnetic ion, is introduced into the system. The impurity modifies the magnetic interaction locally, which makes the ferromagnetic state unstable around the impurity.
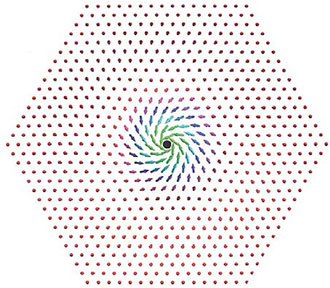
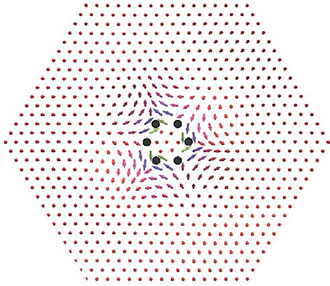
Figure 6. Spontaneous formation of skyrmion-like texture by removing (top) one magnetic ion or (bottom) six neighboring magnetic ions. The defects are represented by black dots.
According to the field theoretical analysis and numerical results, a skyrmion is created around the impurity (Figure 6), which has a lower energy than the ferromagnetic state. The findings suggest a novel mechanism to generate a thermodynamically stable skyrmion in a simple way, which could have a large impact on future applications of skyrmions in spintronic devices, such as memory and magnetic sensors.
Reference: “Magnetic Vortex Induced by Nonmagnetic Impurity in Frustrated Magnets,” Physical Review Letters 116, 187202 (2016); doi: 10.1103/PhysRevLett.116.187202. Authors: Shi-Zeng Lin (Physics of Condensed Matter and Complex Systems, T-4), Saturo Hayami (Hokkaido University, Japan), and Cristian D. Batista (Center for Nonlinear Studies, T-CNLS, University of Tennessee).
Laboratory Directed Research and Development (LDRD) funded the Los Alamos research, and the Institutional Computing Program at LANL provided computer resources for numerical calculations. The work supports the Lab’s Energy Security mission area and the Materials for the Future science pillar through the theoretical design of materials for spintronic devices and other applications. Technical contact: Shi-Zeng Lin