Science Highlights, April 16, 2014
Awards and Recognition







- John Sarrao honored with Ernest O. Lawrence Award
- Mikhail Shashkov named Fellow of the Society for Industrial and Applied Mathematics
- Michael Hamada wins first Gerald Hahn Quality and Productivity Achievement Award
- Postdoc Distinguished Performance Awards honor del Campo, Lanza, and Zheng
- Cory Lueninghoener named conference program chair
Computer, Computational and Statistical Sciences
Field programmable gate array based system deployed to detect smugglers
Materials Physics and Applications
Graphene oxide films developed for a range of technical applications
Awards and Recognition
John Sarrao honored with Ernest O. Lawrence Award
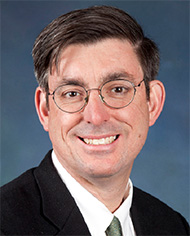
John Sarrao
The DOE has selected John Sarrao (Theory, Simulation and Computation, ADTSC) to receive the 2013 Ernest O. Lawrence Award in Condensed Matter and Materials Sciences. The award recognizes him for his research in plutonium, superconductivity, and magnetism. The citation reads: “For the discovery and study of new materials, especially those based on plutonium, advancing understanding of unconventional magnetic and superconducting states in strongly correlated f-electron condensed matter systems.”
“Actinides, including plutonium, are among the most complex elements and involved in the most complex compounds known to man. Their understanding is fundamental to the Los Alamos core weapons mission and a major challenge in the worldwide condensed-matter and materials communities. John’s contributions have fundamentally advanced our understanding in BOTH of these areas,” said LANL Director Charlie McMillan
Sarrao has been the associate director for Theory, Simulation, and Computation at Los Alamos since March of 2013. He leads the Lab’s efforts in applying science-based prediction to existing and emerging national security missions. Previously, Sarrao was the program director for the Lab’s Office of Science Programs and for the proposed MaRIE (Matter-Radiation Interactions in Extremes) facility designed to provide transformational materials solutions for national security challenges. Sarrao received a PhD in physics from the University of California – Los Angeles for research performed at Los Alamos. He joined the Lab in 1997 as a technical staff member in experimental condensed matter physics. His primary research interest is in the synthesis and characterization of correlated electron systems, especially actinide materials. Sarrao has received the Los Alamos Fellows Prize for Research, in part for his discovery of the first plutonium superconductor. He is a Fellow of the American Association for the Advancement of Science, the American Physical Society, and Los Alamos National Laboratory.
The E.O. Lawrence award was established in 1959 to honor U.S. scientists and engineers at mid-career for exceptional contributions in research and development supporting the DOE and its missions. The award is given for outstanding contributions of exceptionally creative or innovative character. Such achievements (i) must demonstrate significant innovation and discovery; (ii) must be identifiable as transformative; and (iii) must hold prominent scientific or technical leadership. Awardees were chosen in a three-step process: 1) for each award category, a peer review panel assessed the qualifications and merit of all eligible nominees; 2) based on the assessment criteria, the Lawrence Award Program Manager performed an analysis of the peer review findings and made a recommendation, and 3) Energy Secretary Moniz approved the recommendation. The award consists of a gold medal bearing the likeness of E. O. Lawrence, a citation signed by Secretary of Energy Moniz, and an honorarium of $20,000. Technical contact: John SarraoMikhail Shashkov named Fellow of the Society for Industrial and Applied Mathematics
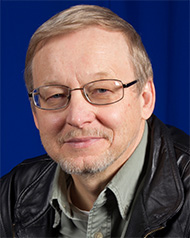
Mikhail Shashkov
The Society for Industrial and Applied Mathematics (SIAM) selected Mikhail (Misha) Shashkov (Methods and Algorithms, XCP-4) as a Fellow. SIAM honored him for his contributions to the development of mimetic finite difference methods for nonlinear systems of partial differential equations. He will be recognized by the professional society at an awards celebration this summer at the SIAM annual meeting in Chicago.
Shashkov joined the Lab’s Theoretical (T) Division in 1994 and moved to X Computational Physics (XCP) Division in 2010, where he leads and develops modern Arbitrary-Lagrangian-Eulerian (ALE) methods for high speed, multi-material flows that are the heart of the Advanced Simulation and Computation (ACS) program for NNSA and LANL weapons calculations. Shashkov holds a doctoral degree in applied mathematics degree from the Keldysh Institute of Applied Mathematics in Moscow, Russia. He also received a Habilitation – highest academic qualification a scholar can achieve by his/her own pursuit earned after obtaining a research doctorate – from Moscow State University. Shashkov became a Los Alamos National Laboratory Fellow in 2012.
SIAM is an international community of more than 13,000 members. Applied mathematics, in partnership with computational science, is essential in solving many real-world problems. Technical contact: Mikhail Shashkov
Michael Hamada wins first Gerald Hahn Quality and Productivity Achievement Award
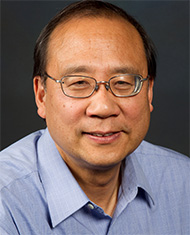
Michael Hamada
The Quality and Productivity (Q&P) Section of the American Statistical Association has chosen Michael Hamada (Statistical Sciences, CCS-6) as the first recipient of its Gerald J. Hahn Q&P Achievement Award. The award recognizes an individual who has demonstrated outstanding and sustained achievement and leadership in developing, promoting, and successfully improving the quality and productivity of products and organizational performance using statistical concepts and methods over a period of 20 or more years.
Hamada will receive the award at the Fall Technical Conference of the Q&P and Physical and Engineering Sciences Sections of ASA and the Chemical and Process Industries and Statistics Divisions of the American Society for Quality. He will deliver the Q&P sponsored plenary address.
Hamada has made extensive contributions to quality and productivity motivated by real-world problems. He received a PhD in statistics from University of Wisconsin – Madison and joined Los Alamos in 1998. Blending his insights gained from experience in government, industry, and academic settings, he has developed statistical solutions for problems of national and international importance while advancing the state of knowledge in the area of quality and productivity. Hamada emphasizes the science behind problems and the understanding of measurement processes, making contributions to reliability, design of experiments, process monitoring, quality improvement, measurement systems, tolerance intervals, sampling, simulation, and uncertainty quantification. Hamada provides innovative, practical solutions, which he communicates to a broad audience and shares the pivotal role that statistics can play in improving engineering and scientific discovery. Hamada is a dedicated mentor and an active collaborator both within his organization and with external colleagues in academic and industrial settings. Technical contact: Michael HamadaPostdoc Distinguished Performance Awards honor del Campo, Lanza, and Zheng
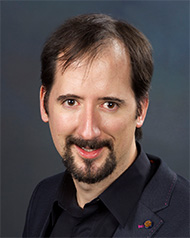
Adolfo del Campo
Three Los Alamos researchers have won Postdoc Distinguished Performance Awards for their research in FY 13. Postdoc Distinguished Performance Awards recognize outstanding and unique contributions by Laboratory postdocs, which results in a positive and significant impact on the Lab’s scientific efforts and status in the scientific community. These awards acknowledge outstanding creativity, innovation, and/or dedication and level of performance substantially beyond that which would normally be expected. The individuals and their research areas are described below. Technical contact: Mary Ann With
Adolfo del Campo (Physics of Condensed Matter and Complex Systems, T-4), a J. Robert Oppenheimer Distinguished Postdoctoral Fellow, is recognized for his outstanding research and leadership in quantum physics and information. He pioneered the field of shortcuts to adiabaticity in many-body systems, with applications in atomic, molecular, and optical physics, and in quantum information. Del Campo designed protocols for ultrafast manipulation of matter-waves and strongly correlated quantum fluids in the laboratory. This work greatly extended the applicability of techniques that have been around for over a decade with only limited applications. He examined fundamental issues in quantum physics and derived a time-energy uncertainty relation for arbitrary physical systems that solved a long-standing issue since the 1940’s. del Campo acted as an Editorial Board member in the Nature Publishing Group (NPG) for Scientific Reports, and he became Review Editor at Frontiers (in partnership with the NPG), in the area of Mathematical Physics. He is the main organizer of the Conference “Shortcuts to Adiabaticity, Optimal Quantum Control, and Thermodynamics,” which brings together international experts in quantum information, statistical mechanics, condensed matter theory, atomic and molecular physics. He is a prolific author of papers in top quality journals, leading theoretical proposals that have produced high impact in the community by prompting new experimental advances. Wojciech Zurek (T-4), and Malcolm Boshier (Applied Modern Physics, P-21) nominated del Campo.
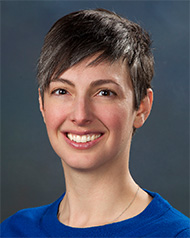
Nina Lanza
Nina Lanza (Space and Remote Sensing, ISR-2), a Postdoctoral Research Associate on the ChemCam team, is recognized for her research to understand the signatures of manganese and rock coatings and rinds on Mars, mission support for ChemCam and the Mars Science Laboratory (MSL), impressive scientific productivity, and support for student research and outreach. A key participant in rapid-turnaround analysis of downlinked ChemCam data and detailed spectral and statistical analyses, she has focused on understanding the signatures of rock coatings and rinds with laser-induced breakdown spectroscopy (LIBS - part of the ChemCam instrument suite). This includes laboratory work on terrestrial samples and observations from Mars. Lanza led the discovery of strong manganese oxide enrichments in various Mars samples. This finding implies that a highly oxidizing environment – much more than needed to simply oxidize iron – must have existed on the Red Planet at some time in the past. By contrast, the Earth’s atmosphere and oceans are thought to have been relatively reducing prior to the production of oxygen by living organisms 2 billion years ago. As a member of the MSL science team involved in instrument development and flight certification, she led the testing of LIBS as a penetration tool for “excavation” beneath the outer coatings on geological samples and completed a set of laboratory studies to prove this capability. This was a major accomplishment for the MSL mission. Lanza has demonstrated a breadth of research that spans fields from geochemistry to geomorphology. She published a chapter on “Extraterrestrial Hillslope Processes” in the multi-volume Treatise on Geomorphology and 16 peer-reviewed papers. She is an enthusiastic presenter of science to outreach groups. Co-mentors, Roger Wiens (ISR-2) and Sam Clegg (Physical Chemistry and Applied Spectroscopy, C-PCS), nominated her.
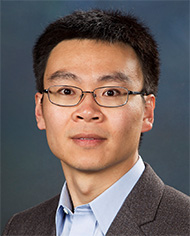
Shijian Zheng
Cory Lueninghoener named conference program chair
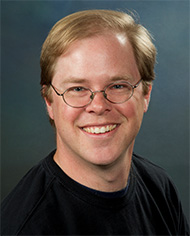
Cory Lueninghoener
Cory Lueninghoener (High Performance Computer Systems, HPC-3) will be the Program Chair of the LISA ’15 (Large Installation System Administration) Conference, which will take place in November of 2015 in Washington, DC. LISA is the premier meeting place for professionals who make computing work across a variety of industries. The conference provides a forum for systems engineering researchers and practitioners to meet and exchange ideas and best practices, covering topics such as systems engineering, security, monitoring, metrics, and culture. System administrators and site reliability engineers attend the conference from research and industry sites around the world, including large web companies, colleges and universities, research laboratories, and small startups. USENIX, the Advanced Computing Systems Association, sponsors the conference. Lueninghoener is also the Workshops Coordinator for LISA ’14, which will be held in Seattle, WA in November of 2014. Technical contact: Cory Lueninghoener
Bioscience
ATHENA surrogate organ system could reduce need for animal drug tests
A multi-institutional collaboration led by Los Alamos is developing a desktop human “body”, called a “homo minutus,” for testing pharmaceuticals and toxic materials. ATHENA, the Advanced Tissue-engineered Human Ectypal Network Analyzer project team, is creating four human organ constructs – liver, heart, lung, and kidney – that are based on a significantly miniaturized platform. Each organ component will be about the size of a smartphone screen, and the whole ATHENA “body” of interconnected organs would fit neatly on a desk. Developing surrogate human organs, coupled with insights from highly sensitive mass spectrometry technologies, could revolutionize the screening of drugs and toxic agents. Rashi Iyer, (Systems Analysis and Surveillance, DSA-3) leads the project, which includes university and industrial collaborators.
The ATHENA project’s goal is to develop a holistic dynamic system that mimics the human physiological environment. The team plans to build a lung that breathes, a heart that pumps, a liver that metabolizes, and a kidney that excretes -– all connected by a tubing infrastructure much akin to the way blood vessels connect our organs. The system would provide understanding of the chemical effects on human organs and could greatly reduce the need for animal or Petri dish testing.
Some 40 percent of pharmaceuticals fail their clinical trials, and there are thousands of chemicals whose effects on humans are unknown. Providing a realistic, cost-effective, and rapid screening system such as ATHENA with high-throughput capabilities could provide major benefits to the medical field, screening more accurately and offering a greater chance of clinical trial success.
Collaborating institutions include Vanderbilt University, Harvard University, University of California – San Francisco, CFD Research Corporation, and Charité Universitätsmedizin, Berlin, Germany. The ATHENA project brings together top capabilities and researchers in this pioneering field of research. The project components are divided as follows:
- In addition to leading and coordinating the project, Iyer is directing work on the lung and kidney organs as well as step-wise integration and validation of all of the organs in the ATHENA perfusion platform – all of which will be executed at LANL.
- John Wikswo (Vanderbilt University) and his team are building the hardware platform that runs and monitors the health of the entire system.
- Katrin Zeilinger (Charité Universitätsmedizin) is developing the first organ, the liver construct.
- Kevin Kit Parker (Harvard University) is leading the heart effort.
- Shuvo Roy (University of California – San Francisco) and William Fissell (Vanderbilt University) are developing the kidney constructs.
- Andrzej Przekwas (CFD Research Corporation) is building a mathematical model of ATHENA to facilitate system design and physiologically-based pharmacokinetic/pharmacodynamic models to guide drug exposure studies and data extrapolation.
- LANL in collaboration with CFD Research Corporation will develop a blood mimic to sustain the four devices.
- The ion mobility mass spectrometry capability being developed by John McLean (Vanderbilt University) with a parallel capability at Los Alamos, led by Srinivas Iyer (Bioenergy and Biome Sciences, B-11), will provide an unprecedented ability to interrogate the system and obtain valuable data.
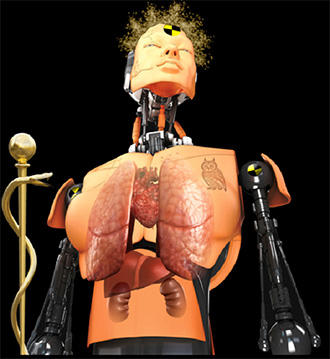
Figure 2. The ATHENA organ project combines heart, liver, kidney and lung features in a desktop toxicity testing platform.
The team has demonstrated successful integration of the Charité-developed liver organ construct into the ATHENA perfusion system. The researchers plan to connect the liver and the heart, followed by the lung and finally the kidney.
In addition to Rashi Iyer and Srinivas Iyer, Los Alamos researchers include Jennifer Harris, Tony Huang, Ayesha Arefin, and Jon Thoma (Biosecurity and Public Health, B-10); Pulak Nath (Applied Modern Physics, P-21); Bob Williams and Tim Sanchez (B-11); and Piyush Bajaj (DSA-3).
The Defense Threat Reduction Agency (DTRA) funds the five-year, $19 million multi-institutional effort. The work supports LANL’s Global Security mission area and the Science of Signatures and Materials for the Future science pillars. Technical contact: Rashi Iyer
Chemistry
Function of ruthenium catalysts in an important class of chemical reactions
Work by Los Alamos researchers published in the Journal of the American Chemical Society proposes a revised mechanism for an industrially important class of catalytic chemical reactions known as the Noyori asymmetric hydrogenation method. Understanding how catalysts function allows scientists to control reaction chemistry and to design more efficient and selective chemical processes. An effective catalyst may facilitate lower temperatures or pressures required in chemical reactions (thus reducing energy input), permit the use of small amounts of added catalyst (important if the catalyst contains a precious metal), and result in more selective production of a desired end-product.
The Noyori method involves the asymmetric hydrogenation of prochiral unsaturated compounds such as ketones, aldehydes and imines. It is one of the most efficient and straightforward methods for preparing optically active compounds for fine chemicals, chiral drug, fragrance, and optical materials applications. The importance of asymmetric hydrogenation resulted in Ryoji Noyori and William Knowles receiving half of the 2001 Nobel Prize in Chemistry. The particular method developed by Noyori uses molecular hydrogen (H2) and small amounts of chiral Ru-BINAP complexes. When introduced it resolved longstanding problems with substrate specificity, unsatisfactory catalytic activity, enantioselectivity, air-sensitivity, etc. The reaction at the heart of the Noyori method has been postulated to proceed via a “metal−ligand bifunctional mechanism,” a theory that has directly influenced the appearance of a powerful new branch of homogeneous catalysis, called bifunctional molecular catalysis.
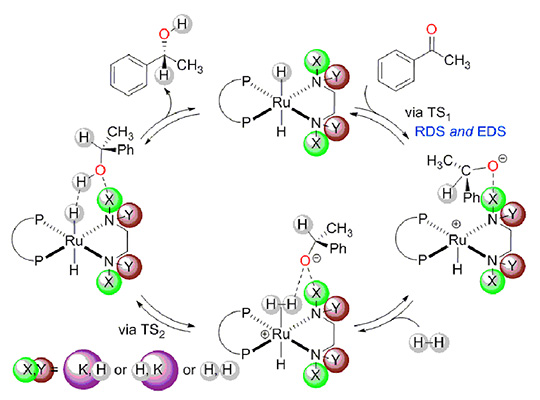
Figure 1. The revised catalytic cycle for the hydrogenation of acetophenone proposed by the authors of the paper. RDS is rate determining step; EDS is enantiodetermining step; TS is transition state.
A desire to gain a deeper understanding of the reaction mechanism prompted the LANL researchers to re-investigate the Noyori hydrogenation reaction through experimental and computational methods. Their results indicate the need for important revisions to the current theory. The team demonstrated that the reaction did not conform as predicted to several experimental observations. The scientists suggested a different catalytic cycle for hydrogenation of acetophenone by Noyori’s catalyst. This finding changes our understanding of how metal-ligand bifunctional mechanisms may occur (Figure 1 scheme). Research in this area has been driven by demands for more powerful and sophisticated catalysts with tunable functions, and a clearer understanding of the underlying chemistry is required to achieve these ends.
Reference: “Unravelling the Mechanism of the Asymmetric Hydrogenation of Acetophenone by [RuX2(diphosphine)(1,2-diamine)] Catalysts,” Journal of the American Chemical Society 136, 3505 (2014); doi: 10.1021/ja411374j). Co-authors are Pavel Dub (Inorganic, Isotope and Actinide Chemistry, C-IIAC), Neil Henson and Rich Martin (Physics and Chemistry of Materials, T-1), and John Gordon (C-IIAC).
The Laboratory Directed Research and Development (LDRD) Program funded this work, which supports the Lab’s Energy Security mission area through more efficient catalysis and the Materials for the Future science pillar. Technical contact: John Gordon
Computer, Computational and Statistical Sciences
Field programmable gate array based system deployed to detect smugglers
Los Alamos researchers deployed a field-programmable gate array (FPGA)-based phase interferometry system to the Smithsonian Observatory at Mt. Hopkins, outside of Tucson, AZ. The deployment is a proof of concept test to demonstrate direction finding and single-platform geo-location from 5-500 MHz for the Department of Homeland Security’s Customs and Border Protection Program. This is a true “field-programmable” system wherein scientists can update firmware and software at will, remotely. The Los Alamos team designed antennas, RF filters, signal processing algorithms, and computational support. The scientists created the system to help find drug and human traffickers near the Mexican border. The ability to use a single platform part is important because the system is meant to be on a plane. Thus, all of the antennas are close together and moving.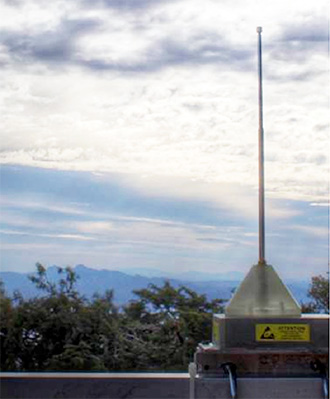
Photo. The field programmable gate array deployed on Mt. Hopkins.
The technology detects radio signals from push-to-talk radios (walkie-talkies) across the 1-500 Mhz band. The ability to geo-locate the signals and identify the signal types provides information regarding the likelihood that the radio operators are smugglers/traffickers of drugs or humans. For instance, truckers on CB radios on the freeway are not likely to be nefarious actors, but encrypted radios way in remote areas suggest the possibility of people concealing their activities. The underlying mathematics involves simultaneous 64 kilo-point Fast Fourier Transforms (FFTs) that provide instantaneous measurement of the difference between signal arrival times at multiple antennas. The FFT converts a series of measurements of the voltage from the antenna into the frequency domain. Instead of the points making up a sine wave, the magnitude and phase of that sine wave can be obtained. This effectively separates the combination of all of the radio channels in the sky that arrives at the antenna into individual channels.
Zachary Baker (Applied Computer Science, CCS-7) led the research team of Mark Dunham (Emerging Threats, GS-ET), Michael Caffrey (Space Data Systems, ISR-3), Joseph Palmer, Michael Pigue, and Paul Snow (Space Electronics and Signal Processing, ISR-4); Bobby Quintana (Space Instrumentation, ISR-5); and Bryan Haynes (RF Engineering, AOT-RFE). Homeland Security’s Customs and Border Protection Program funded the work, which supported the Lab’s Global Security mission area and the Information, Science, and Technology and Science of Signatures science pillars. Technical contact: Zachary Baker
LANSCE
Measuring the neutron capture cross-section of plutonium-239
The plutonium-239 (239Pu) neutron capture cross-section is an important factor used in many reactor calculations and in weapons-related calculations. The Advanced Reactor Concept program, a DOE program considering the next generation of reactor designs, identified a need for precise knowledge of the 239Pu cross section above 2 keV. A collaboration of researchers in LANL’s Neutron and Nuclear Science (LANSCE-NS) and Nuclear and Radiochemistry (C-NR) groups, and Lawrence Livermore National Laboratory have now measured the cross-section. Physical Review C has published the findings.
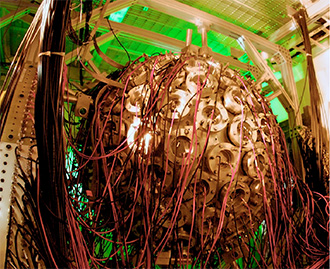
Photo. DANCE.
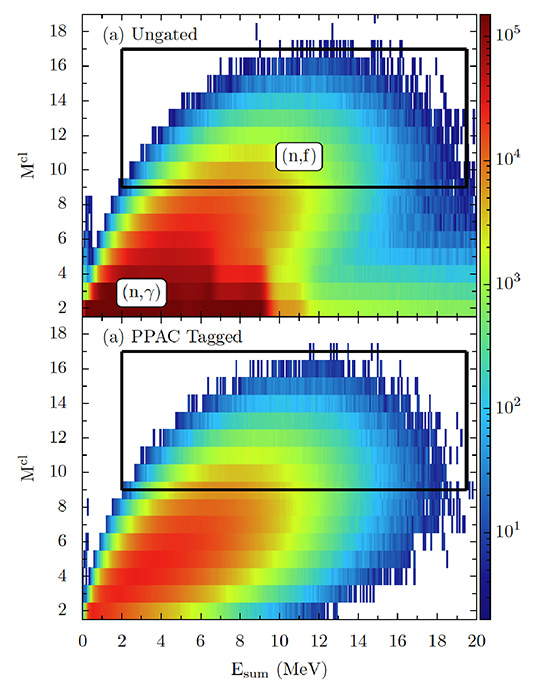
Figure 3. Mcl vs. Esum for ungated (a) and PPAC coincidence (b). The box defines a region where only fission contributes to the spectrum, and is used to determine the PPAC efficiency.
Results of the 239Pu capture-to-fission ratio α and the 239Pu(n,γ) cross section deduced for the neutron energy region 10 eV to 1 keV have been determined. This measurement largely confirms the current evaluation, but differences in the individual resonances strengths occur in several cases. The present work represents a significant advancement in experimental technique compared with prior work. The detailed background suppression and subtraction methods result in a marked improvement for weak signal-to-noise regions. The researchers explored the signature of fission events in DANCE via measurements from gamma rays emitted in coincidence with a PPAC signal triggered by a fission fragment. The result enables possible future work to extend the measurement to higher neutron energies.
Reference: “Improved Neutron Capture Cross Section of 239Pu,” Physical Review C 89, 034610 (2014); doi: 10.1103/PhysRevC.89.034610. Researchers include Shea Mosby, Andrii Chyzh, Aaron Couture, John O’Donnell and John Ullman (LANSCE-NS); Todd Bredeweg and Marian Jandel (C-NR); R. Henderson, E. Kwan, and C. Y. Wu (Lawrence Livermore National Laboratory).
NNSA-NN funds the work, which supports LANL’s Nuclear Deterrence and Energy Security mission areas and the Nuclear and Particle Futures science pillar. Technical contact: Shea Mosby
Materials Physics and Applications
Graphene oxide films developed for a range of technical applications
In two recent publications, Los Alamos researchers and collaborators examine graphene oxide films. These films have applications for a range of technologies, including energy, catalysis, sensing, and gas/moisture barriers. The research is described in the following sections.
Building reduced graphene oxide thin films as ultrabarriers for organic electronics
In Advanced Energy Materials, Materials Synthesis and Integrated Devices (MPA-11) scientists working with collaborators from Rutgers University, Georgia Institute of Technology, and Tohoku University (Japan) report that properly stacking atomically thin sheets of carbon, specifically graphene oxide, leads to bulk thin films that prevent oxygen/moisture penetration better than current commercially available polymer-chain based barrier films. The results provide a new route for the design and preparation of high-performance and solution-processable gas/moisture barriers, which have potential applications for a wide range of encapsulation technologies.
Taking advantage of the unique properties of graphene, which prevents even the smallest atoms of hydrogen to pass due to its close-packed hexagonal structure, the team developed a scalable chemical route to produce the reduced graphene oxide material. The researchers filtered the material to form thin films. They applied their barrier films to working organic photovoltaic devices (OPVs) and monitored device performance over time. The team chose OPVs due to their high sensitivity to environmental conditions (i.e., oxygen and moisture levels). The new films made by this process resulted in substantial improvements in OPV lifetime relative to devices without a barrier layer as well as devices encapsulated in commercially available polymer-chain-based barrier films.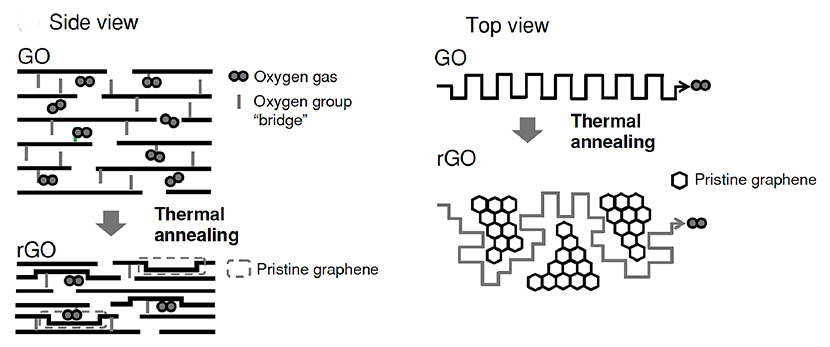
Figure 4. (Left): Schematics of side view showing oxygen gas permeating through graphene oxide, GO (top) and reduced graphene oxide, rGO (bottom). For the case of GO, oxygen molecules permeates through the interlayer due to presence of oxygen functional groups acting as “bridges”. However, pristine graphene nanoislands created by removal of oxygen group “bridges” clog permeation paths (dashed boxes), increasing the diffusion length as illustrated in top view. (Right): Possible permeation paths of the oxygen are indicated by the black and gray arrows for GO and rGO, respectively.
Reference: “Reduced Graphene Oxide Thin Films as Ultrabarriers for Organic Electronics,” Advanced Energy Materials 4, 1300986 (2014); doi: 10.1002/aenm.201300986. Authors include Hisato Yamaguchi (MPA-11 and Rutgers University), Wanyi Nie, Gautam Gupta, and Aditya Mohite (MPA-11); Jimmy Granstrom, Hossein Sojoudi, and Samuel Graham (Georgia Institute of Technology); Takeshi Fujita and Mingwei Chen (Tohoku University, Japan); Damien Voiry and Manish Chhowalla (Rutgers University).
A Laboratory Directed Research and Development (LDRD) program Director’s Postdoctoral Fellowship funded the Los Alamos portion of the work. The research supports the Laboratory’s Energy Security mission area and Materials for the Future science pillar. Technical contact: Aditya Mohite
Ozonated graphene oxide film as a proton-exchange membrane
Soon after the discovery of graphene, GO began attracting considerable interest because of its unique electronic and optical properties. Graphene oxide contains several chemical functional groups that are attached to the graphite basal plane and can be manipulated to tailor GO for specific applications. The new study demonstrates that modifying dispersed GO presents a powerful opportunity to optimize a nanoscale material for proton exchange membranes. The researchers revealed that the reaction of GO with ozone results in a high level of oxidation, which leads to significantly improved ionic (protonic) conductivity of the GO. The team synthesized freestanding ozonated GO films and used them as efficient proton-conducting electrolytes for fuel cell membranes. The increase in protonic conductivity of the ozonated GO originates from enhanced proton hopping, which is due to the higher content of oxygenated functional groups in the basal planes and edges of ozonated GO as well as the morphological changes in GO caused by ozonation.
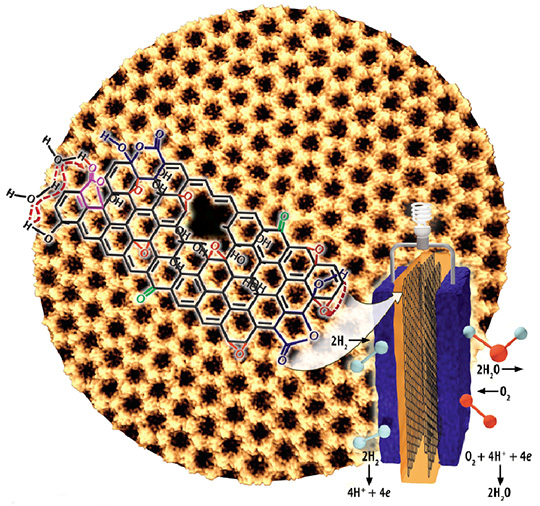
Figure 4. Showcased
Reference: “Ozonated Graphene Oxide Film as a Proton-Exchange Membrane,” Angewandte Chemie International Edition 53, 3588 (2014); doi: 10.1002/anie.201310908. The lead author is Director’s Postdoctoral Fellow Wei Gao. Coauthors include W. Gao, G. Wu, R. Mukundan, E. L. Brosha, A. M. Dattelbaum, and P. Zelenay (Materials Synthesis and Integrated Devices, MPA-11); J. K. Baldwin (Center for Integrated Nanotechnologies, MPA-CINT); M.T. Janicke; D.A. Cullen, and K.L. More (Oak Ridge National Laboratory); C. Galande and P.M. Ajayan (Rice University).
Funding for the Los Alamos work came from the DOE Office of Energy Efficiency and Renewable Energy through the Fuel Cell Technologies Office and from Los Alamos National Laboratory through the Laboratory Directed Research and Development (LDRD) program and a Director’s Postdoctoral Fellowship. The work was conducted in part at the Center for Integrated Nanotechnologies (CINT), a DOE Office of Science User Facility operated by Los Alamos National Laboratory and Sandia National Laboratories. The research supports the Lab’s Energy Security mission area and Materials for the Future science pillar. Technical contact: Piotr Zelenay
Materials Science and Technology
Examining the mechanical response in cellular materials
Understanding how material composition, geometry, aging, and processing affect the overall material performance in the nation’s stockpile requires a detailed understanding of the initial morphology and how the morphology changes under external stimuli. A laboratory based X-ray computed tomography (CT) system can image polymer foams as they are compressed in an interrupted in situ modality. Due to the low flux of a laboratory X-ray source when compared with the synchrotron, stress relaxation must occur in the material before a tomogram can be collected. Otherwise, the residual sample motion leads to image blur. This relaxation process requires approximately 20 minutes before the tomogram can be collected over more than one hour. Important information regarding the compressive performance and morphology changes is lost during this stress relaxation time (Figure 5, black box).
Third generation synchrotron light sources, such as the Advanced Photon Source (APS) at Argonne National Laboratory, afford materials scientists unprecedented X-ray flux to help solve complex materials science challenges. Coupling this high flux with a high-speed camera provides X-ray radiographs every millisecond and generates tomographic data in ~1 second and ~5 μm voxel size. Adding a sample compression cell makes it possible to study the dynamic in situ deformation of polymeric foams at a 10-2 strain rate. LANL researchers and collaborators imaged a variety of cellular foam materials at the APS synchrotron to capture the morphological changes in polymeric foam materials during dynamic compression. The team acquired 20 full tomograms of each sample while simultaneously compressing the samples up to ~60%, all within a total experiment time of 100 seconds. The scientists examined hydrogen blown silicone foams (LK3626, SX358 exposed to various aging conditions), pelletized urea silicone foam, syntactic Sylgard-184, and additively manufactured foams.
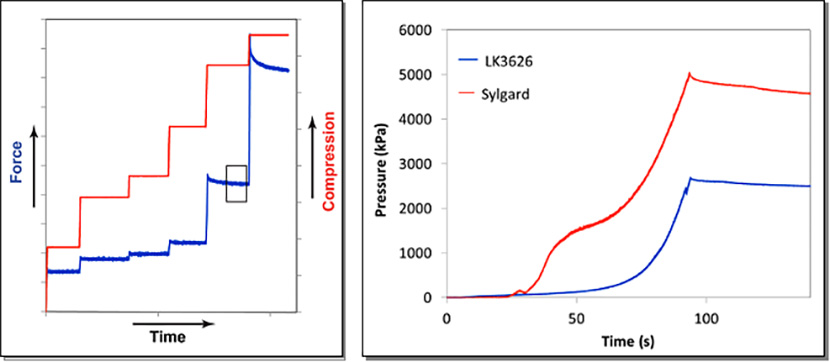
Figure 5. (Left): Typical interrupted in situ force and compression curve for a foam in a laboratory setting. Laboratory systems must have stable samples and require a longer period of time to image. Imaging must occur after stress relaxation (black box). (Right): Force curves for two foams, LK3626 and syntactic Sylgard, as they are continuously compressed at the synchrotron. Compression and imaging for these experiments halts at a maximum force at ~100 seconds.
The researchers measured and correlated the differences in mechanical performance of polymeric foams to their physical/morphological changes with this technique. For example, LK3626 is a soft-structured hydrogen blown silicone foam. It has a weak ligament structure that quickly proceeds directly to buckling with almost no bending resistive force (Figure 5, right). Figure 6 reveals almost no Poisson effect. Reinforcement from 100-μm glass microballoons makes syntactic Sylgard 184 a much stiffer material. Therefore, Sylgard exhibits a stress/strain curve with definitive bending/ buckling/ compressing regions (Figure 5, right and Figure 7). Acquiring the data continuously, with no pause for stress relaxation or prolonged imaging times, allows for a much simpler mechanical interpretation of the experiments.
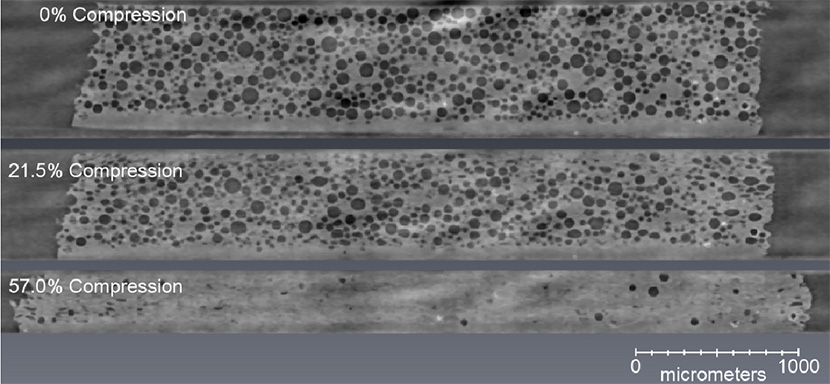
Figure 6. Compression of LK3626 hydrogen-blown foam. The sample is completely compressible with little Poisson effect. Ligaments can be seen breaking with material snapping back into place as it is compressed. Figure 7. Compression of syntactic Sylgard foam with 100 μm hollow glass beads. The sample is nearly incompressible and displays a high Poisson ratio.
Physics
Proton radiography studies ejecta-sheet transport
Modeling the formation of micron and submicron material ejected from a shocked metal interface is a current focus of the NNSA Advanced Simulation and Computing (ASC) Physics and Engineering Models Mix program. The complex physics of this process depends on the nature of the metal released state (solid, fluid or mixed-phase), the nature of the second medium (vacuum or shocked gas), and the geometry of the interface perturbations. Proton Radiography (pRad) provides an exquisite microscope for observing the temporal evolution of such perturbed interfaces from the incidence of a shockwave through the growth of surface Richtmyer-Meshkov instabilities (RMI) to their breakup and subsequent evolution. Los Alamos researchers have conducted two shockwave pRad experiments that provided detailed information for current models of breakup when an initial tin surface, characterized by two-dimensional sinusoidal perturbations, was shocked to first shockwave loading pressures of order 24 GPa.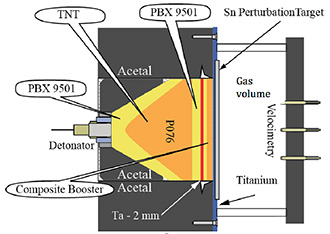
Figure 8. The FY14 pRad Eve physics package. The package encases in acetal the high explosive lens, anvil and booster systems. The shockwave reflected from the Sn target system (Ti + Sn) reflects back to the anvil, which reflects the shockwave to the Sn target system to cause the second shockwave.
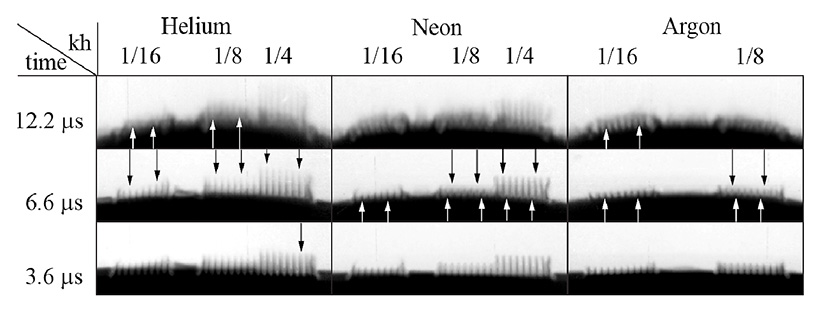
Figure 9. Eve experiments: 2-shock RMI sheet transport at pRad. The lower images show only first-shock RMI (the second shock occurs at ~4 s). In the subsequent images, the white arrows indicate locations of RMI subsequent to the second shock (where we see them), and the black arrows indicate locations of the first shock instabilities.
The images shown in Figure 9 validate the gas density dependence in this model for tin/helium, tin/neon, and tin/argon interfaces. The unperturbed flat interfaces between the perturbed regions eject quite a lot of mass at the second shock, and the perturbations break up quickest in argon, then neon, and then helium. The details of shock growth provide critical data for validation of multi-shock ejecta models.
The Advanced Simulation and Computing (ASC) program is a DOE/NNSA computational/multi-physics program whose goal is predictive stockpile stewardship in the absence of nuclear testing. ASC is a collaborative program among Lawrence Livermore, Los Alamos, and Sandia national laboratories to ensure the safety and reliability of the nation's nuclear weapons stockpile.
William T. Buttler (Neutron Science and Technology, P-23), James E. Hammerberg (Materials and Physical Data, XCP-5), David M. Oro (P-23) and the pRad Team conducted the experiments. Derek Schmidt, John Martinez, and Kimberly Obrey (Polymers and Coatings, MST-7) fabricated the experimental targets. NNSA Science Campaign 1 (Primary Assessment Technologies) funded the pRad experiments, which support the Lab’s Nuclear Deterrence mission area and Nuclear and Particle Futures and Information, Science, and Technology science pillars. Technical contact: William Buttler