Science Highlights, October 25, 2017
Awards and Recognition
Earth and Environmental Sciences
Predicting first-passage times in sparse discrete fracture networks
Explosive Science and Shock Physics
X-ray laser experiments show that scientists have overestimated meteor sizes
Materials Physics and Applications
Atomic-level observations reveal how low-cost fuel cell catalysts work
Nuclear Engineering and Nonproliferation
Advanced formalism enhances neutron counting capability for nuclear safeguards
Theoretical
Emergent Hall physics in 2-D quantum materials combines Hall effects of matter and light
Awards and Recognition
Aditya Mohite named 2017 Resonate Award winner
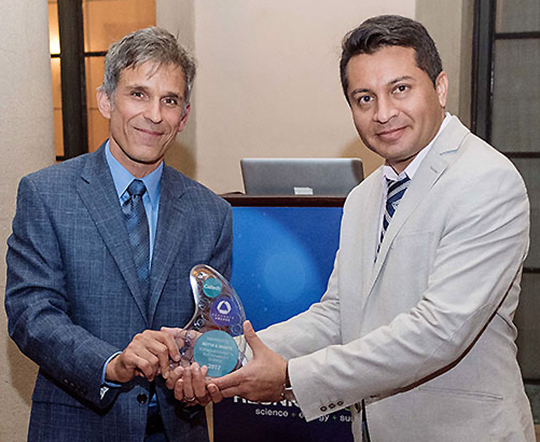
Aditya Mohite (right) accepts the 2017 Resonate Award from Resnick Institute Director Jonas Peters.
The faculty in the California Institute of Technology, Resnick Sustainability Institute presented the 2017 Resonate Award to Aditya Mohite (Materials Synthesis and Integrated Devices, MPA-11). The award cited him for developing two-dimensional and thin-film materials for high-performance solar electricity generation.
The Resonate Award honors outstanding achievement in renewable energy and sustainability-focused science and technology. It aims to highlight promising innovators who have made new contributions to sustainability science, particularly in energy security, climate change, and the environment.
Mohite earned a Ph.D. in electrical engineering from The University of Louisville. He joined the Laboratory in 2009 as a postdoctoral researcher in the Center for Integrated Nanotechnologies (MPA-CINT) and became a staff scientist in 2011. Mohite, an expert in device physics and material science, directs an energy and optoelectronic devices lab and leads the Light to Energy team. His research focuses on understanding and controlling charge and energy transfer processes occurring at interfaces created with organic and inorganic materials for thin-film clean energy technologies. Achieving this requires an in-depth understanding and control of physical properties across multiple scales at relevant operating conditions. These strategies have translated to high performance devices with the stability required to be useful in the real world and more than 100 peer-reviewed papers. Mohite’s research supports the Laboratory’s Energy Security mission area and its Materials for the Future science pillar through the development of materials for solar energy generation.
The Resnick Sustainability Institute draws on the California Institute of Technology’s legacy of innovation, seeking to empower next-generation pioneers by recognizing their contributions to sustainability across all sciences and technology. The Resnick Institute is dedicated to supporting the cutting-edge science and creative problem solving necessary to change the balance of the world’s sustainability.
Mohite received the award at a special reception as part of the school’s 2017 Resnick Young Investigators Symposium, which celebrates young researchers who have established science and engineering initiatives that show promise in tackling sustainable future challenges. Technical contact: Aditya Mohite
Eight Laboratory innovations honored with R&D 100 Awards
Eight Los Alamos National Laboratory technologies won R&D 100 Awards at R&D Magazine’s annual ceremony in Orlando, FL. In addition, EDGE Bioinformatics also won a Special Recognition Award in the Market Disruptor-Products Category. The prestigious “Oscars of Invention” honor the top 100 proven technological advances of the past year as determined by a panel selected by R&D Magazine. The R&D 100 Awards span industry, academia and government-sponsored research organizations. The Awards, with projects covering energy, modeling and simulation, health, materials, and engineering, demonstrate the continued success of Laboratory researchers in technical innovation for national security science. Since 1978 Los Alamos has won 145 of the R&D 100 Awards. Technical contact: Janet Mercer-Smith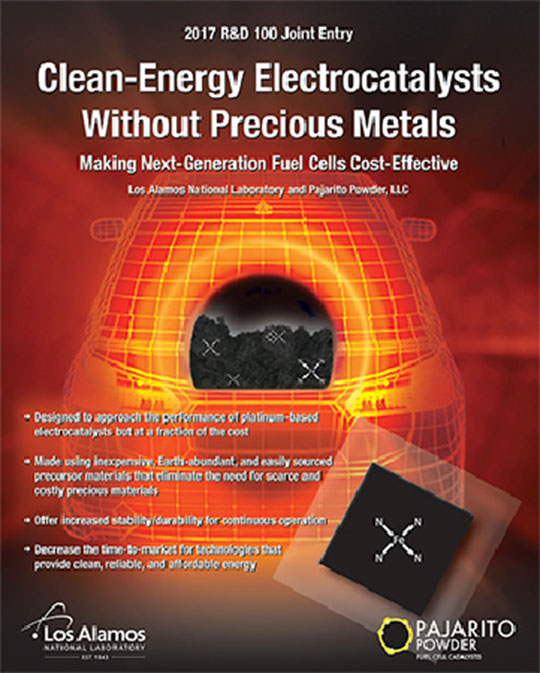
Clean-Energy Catalysts Without Precious Metals use inexpensive, Earth-abundant, and easily sourced precursor materials for the synthesis of electrocatalysts for hydrogen-based fuel cells. The fuel cells produce electrical energy and emit one byproduct: water. Conventional fuel cells rely on costly precious metal catalysts – such as platinum. The new precious-metal-free electrocatalysts generate performance approaching that of precious metal catalysts but at a fraction of the cost. Moreover, these new catalysts have a much higher tolerance to virtually all contaminants and impurities commonly present in the fuel cell hardware, hydrogen, and air feed stream. Potential applications include transportation, portable batteries, and stationary emergency backup power.
Los Alamos submitted the joint entry with Pajarito Powder, LLC based on technology that Pajarito Powder licensed from the Lab. Piotr Zelenay (Materials Synthesis and Integrated Devices, MPA-11) led the Los Alamos team of Hoon Taek Chung, Edward Holby, and Ulises Martinez. Pajarito Powder collaborators include Barr Zulevi, Alia Lubers, Geoff McCool, and Sam McKinney.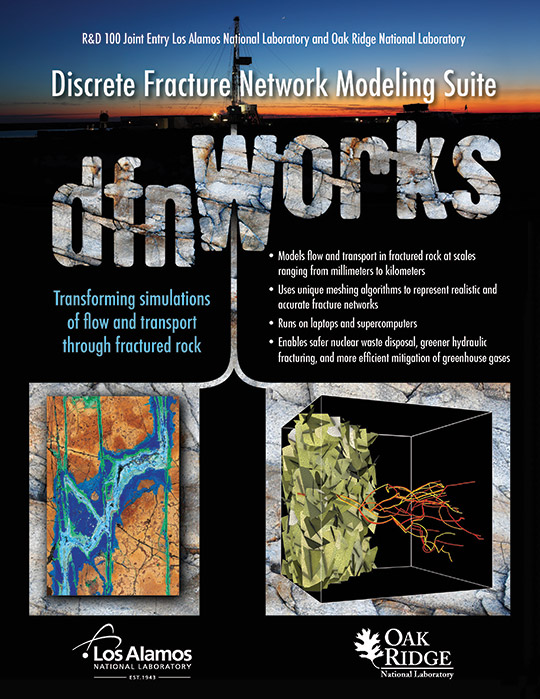
dfnWorks: Discrete Fracture Network Modeling Suite is a computational suite that simulates and predicts the flow and transport of fluids through underground fractured rock. It covers length scales that range from millimeters to kilometers, can run on computers as small as a laptop and as large as a supercomputer, and requires minimal effort to create representative models. Applications for dfnWorks include helping catch rogue nations performing clandestine underground nuclear tests, maximizing the extraction of natural gas, oil, and geothermal wells while minimizing environmental impacts; helping scientists design effective methods for effective carbon sequestration to slow the accumulation of greenhouse gases; and aiding the development of methods to ensure the safe disposal of nuclear waste in underground repositories. Link to video.
Los Alamos submitted the joint entry with Oak Ridge National Laboratory. Carl Gable led the team of Jeffrey Hyman, Satish Karra, Nataliia Makedonska, and Hari Viswanathan (Computational Earth Science, EES-16). Scott Painter (Oak Ridge National Laboratory) collaborated.
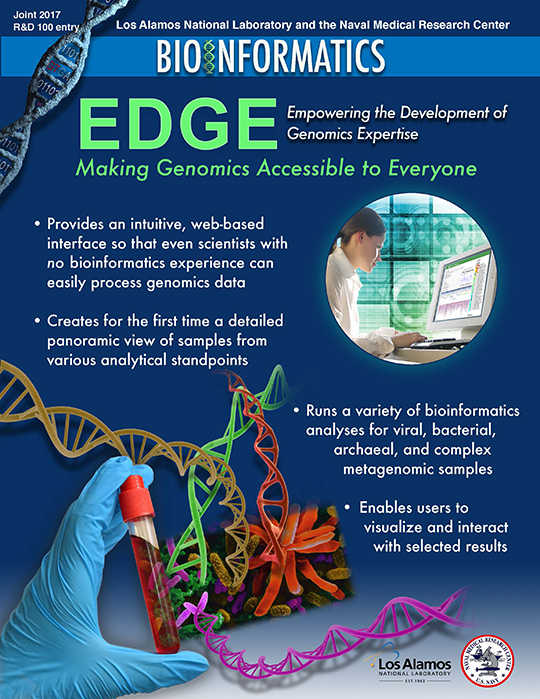
EDGE (Empowering the Development of Genomics Expertise) Bioinformatics “democratizes” the genomics revolution by enabling any researcher or physician to analyze complex genomics data quickly and easily. The intuitive, web-based platform can be applied to a wide variety of genome-sequencing samples ranging from individual isolates (from a culture of a single organism) to much more complex metagenomics (microbiome) projects. The platform addresses the problem of handling Big Data, without users having to possess bioinformatics expertise. EDGE brings the power of complex, big-data sequencing analysis to smaller research laboratories, including clinics, hospitals, universities, and remote sites.
Los Alamos submitted EDGE as a joint entry with the Naval Medical Research Center. Patrick Chain led the Los Alamos team of Po-E Li, Chien-Chi Lo, Karen Davenport, Yan Xu, Pavel Senin, and Migun Shakya (Biosecurity and Public Health, B-10). Collaborators at the Naval Medical Center include Theron Hamilton, Kimberly Bishop-Lilly, Joseph Anderson, Logan Voegtly, and Casandra Philipson.
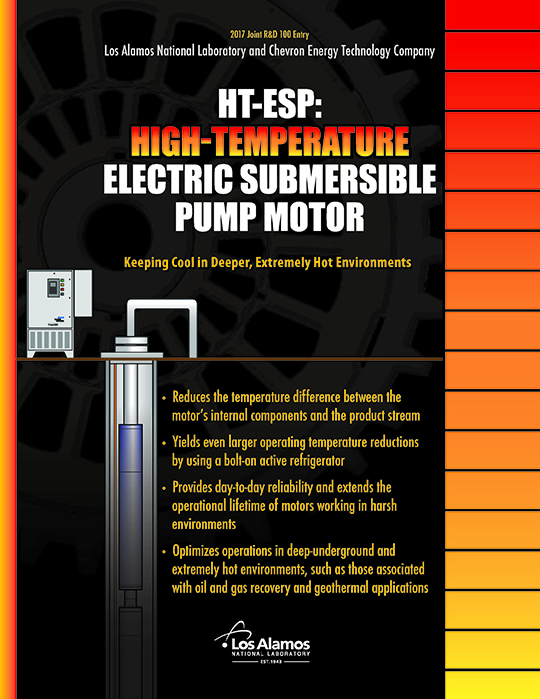
High-Temperature Electric Submersible Pump Motor (HT-ESP) is rugged and reliable, offering improved thermal performance compared with conventional submersible pumps used in deep underground and extremely hot environments. Whether it’s drilling deeper for oil and gas reservoirs or tapping into geothermal resources of energy, today’s electric submersible pump motors must operate in some harsh, extremely hot environments. Current pump motors overheat and must be replaced often. Therefore, the Los Alamos/Chevron research team developed two technologies for HT-ESP to lower the internal operating temperature of the motor: 1) passive heat-transfer enhancements to the motor and 2) a bolt-on active onboard refrigerator. The HT-ESP motor provides operational reliability, thus extending the operational lifetime of motors working in deep underground and extremely hot environments. Moreover,the HT-ESP motor increases its operational range, enabling it to stay cool in higher temperature environments where conventional motors suffer from frequent failures associated with overheating.
Los Alamos submitted the High-Temperature Electric Submersible Pump Motor as a joint entry with Chevron Energy Technology Company. Todd Jankowski (Mechanical and Thermal Engineering, AET-1) led a Los Alamos team of Dallas Hill, Britton Lambson, James Stewart, Robert Bourque, and Coyne Prenger. Chevron collaborators include Jose Gamboa, Daniel Hunt, Max Bough, and Yamila Orrego.
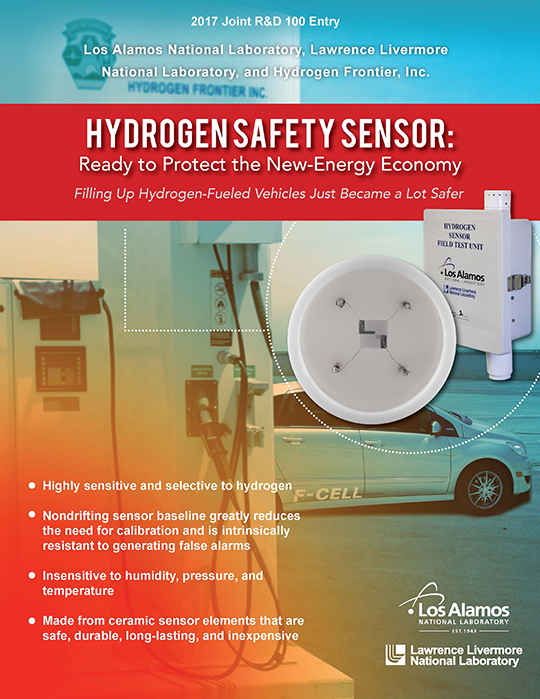
Hydrogen Safety Sensor makes filling up hydrogen-fueled vehicles a lot safer. Hydrogen gas is highly flammable, colorless, odorless, and propagates very quickly if released into the air. It is not possible to introduce odorants to detect the presence of hydrogen leaks because the chemicals used to create a foul warning odor would damage the catalysts and internal components of the vehicle’s fuel-cell stack. The Hydrogen Safety Sensor is made of safe, durable, and long-lasting ceramic sensor elements of the type used in automotive oxygen sensor technology. The safety sensors can be placed anywhere in the hydrogen supply chain, from hydrogen production and distributions to a critical component of the hydrogen “pump” at a filling station to a functioning safety component of the consumer product itself. Testing at Hydrogen Frontier filling stations has demonstrated that the sensors are highly sensitive and selective to hydrogen with no baseline drift or false alarms. All facets of the hydrogen economy, from transportation to hydrogen generators to off grid power sources, could benefit from the hydrogen safety sensors.
Los Alamos submitted the Hydrogen Safety Sensor as a joint entry with Lawrence Livermore National Laboratory and Hydrogen Frontier, Inc. Eric Brosha led the Los Alamos team of Christopher Romero, Rangachary Mukundan, and Cortney Kreller (Materials Synthesis and Integrated Devices, MPA-11). Collaborators include Amanda Wu and Robert Glass (Lawrence Livermore National Laboratory) and Daniel A. Poppe (Hydrogen Frontier, Inc.).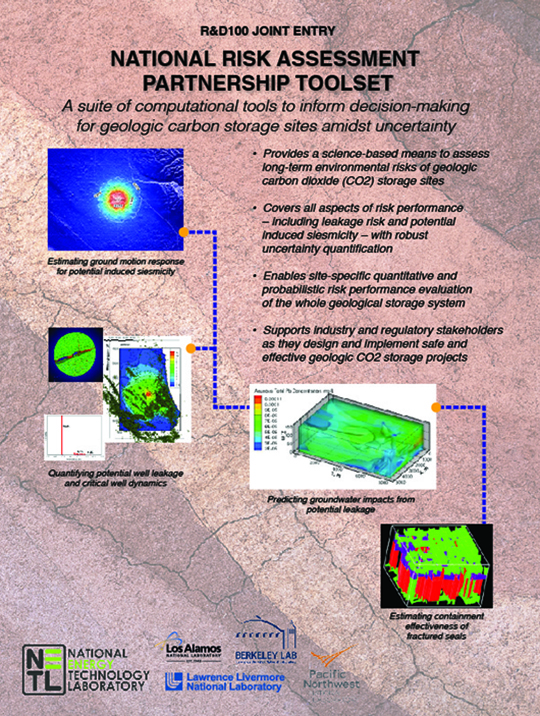
National Risk Assessment Partnership Toolset is a set of ten science-based computational tools developed to assess long-term environmental risks of geologic carbon dioxide (CO2) storage sites. This novel toolset is the only product suite that allows rapid, site-specific quantitative and probabilistic risk performance evaluation of the whole geological CO2 storage system – from storage reservoir to overlying groundwater and the atmosphere. These tools support industry and regulatory stakeholders as they design and implement safe and effective geological CO2 storage projects to sequester large volumes of human-made CO2. Accessed by over 250 stakeholders from academia, regulatory agencies, and industry, the NRAP Toolset is now in use by the carbon storage community across the world, including projects in Asia and Australia.
The National Energy Technology Laboratory submitted the joint entry with Los Alamos National Laboratory, Lawrence Berkeley National Laboratory, Lawrence Livermore National Laboratory, and Pacific Northwest National Laboratory. Rajesh Pawar (Computational Earth Science, EES-16) led the Los Alamos team of Chris Bradley (Geophysics, EES-17), Elizabeth Keating, Phil Stauffer, Shaoping Chu, and Dylan Harp (EES-16); Richard Lee (Process Modeling and Analysis, AET-2); Bill Carey (Earth System Observations, EES-14); and George Guthrie (Earth and Environmental Sciences, EES-DO).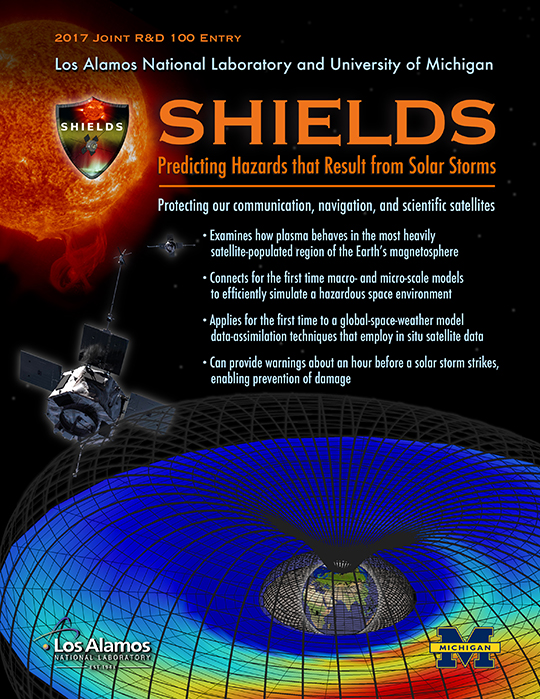
SHIELDS (Space Hazards Induced near Earth by Large, Dynamic Storms) protects communication, navigation, and scientific satellites orbiting the Earth’s magnetosphere by predicting hazards resulting from solar storms that cause “space weather”. The space weather could damage onboard electronics in satellites and thus interrupt radio and television reception, disrupt the operation of cellphones and GPS, shut down the Internet, and endanger military and civilian operations. Researchers developed the software platform to understand, model, and predict this weather about an hour before it hits satellites, enabling instruments to be placed in a safe mode. Multiscale modeling and satellite observational data create an end-to-end model of the magnetosphere driven by the dynamic solar wind. A thorough understanding of space weather and spacecraft charging is needed to strengthen spacecraft design and mitigate these hazards. One component of SHIELDS is already operational at the National Oceanic and Atmospheric Administration’s Space Weather Prediction Center to provide short-term forecasts and enable operations to prevent damage. Link to video.
Los Alamos submitted SHIELDS as a joint entry with the University of Michigan. Vania Jordanova (Space Science and Applications, ISR-1) led the Los Alamos team of Gian Luca Delzanno, Humberto Godinez, J. David Moulton, and Daniil Svyatsky (Applied Mathematics and Plasma Physics, T-5); Michael Henderson, Steve Morley, Jesse Woodroffe, and Thiago Brito (ISR-1); Christopher Jeffery, Alin-Daniel Panaitescu, and Collin Meierbachtol (Space and Remote Sensing, ISR-2); Earl Lawrence (Statistical Sciences, CCS-6); and Louis Vernon (Applied Computer Science, CCS-7). University of Michigan collaborators included Gabor Toth, Daniel Welling, Yuxi Chen, and John Haiducek.
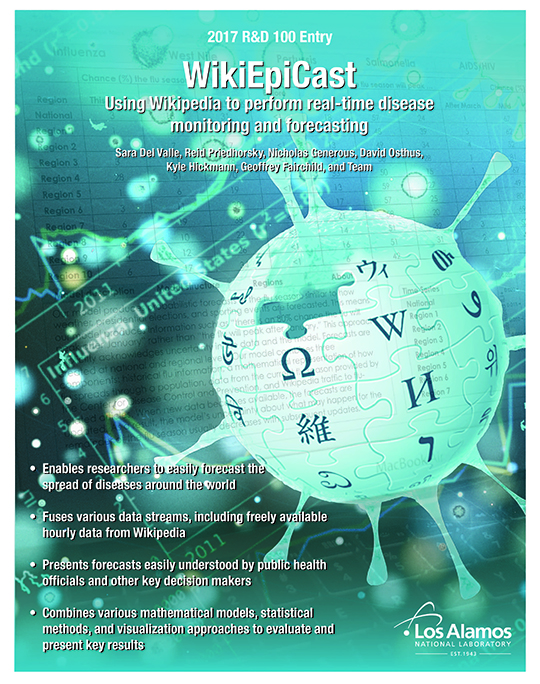
WikiEpiCast combines mathematical models with clinical surveillance data and readership traffic from Wikipedia to forecast the spread and severity of diseases around the world. Successfully demonstrated on forecasting influenza in the United States, WikiEpiCast’s framework can be applied to any communicable disease, from Ebola and tuberculosis to Zika virus.
The tools being developed within WikiEpiCast present probabilistic forecasts, similar to how the nightly newscasts present weather updates. As a result, such forecasts are easy for nonscientist decision makers to digest and in turn make informed decisions that could save lives and potentially mitigate the potential impacts of an epidemic or pandemic of a burgeoning communicable disease.
Los Alamos submitted the WikiEpiCast entry. Sara del Valle (Information Systems and Modeling, A-1) led the team of Nicholas Generous and Geoffrey Fairchild (A-1), Kyle Hickmann (Verification and Analysis, XCP-8), Reid Priedhorsky (High Performance Computing Environments, HPC-ENV), and David Osthus (Statistical Sciences, CCS-6).
Xu, Meyers, Chamberlin, Leibman, Yoshida, Sansinena win NNSA Sustainability Award
Researchers Ning Xu, Lisa Meyers, Rebecca Chamberlin (Actinide Analytical Chemistry, C-AAC); Chris Leibman (Materials Synthesis and Integrated Devices, MPA-11); and Tom Yoshida and Jose-Maria Sansinena (Chemical Diagnostics and Engineering, C-CDE) received the 2017 NNSA Sustainability Award in the Waste Reduction and Pollution Prevention category for their work to replace hazardous chemicals during material processing with less hazardous ones. The team was honored for the achievement of Dissolving uranium oxide with ammonium bifluoride. NNSA Deputy Associate Administrator for Enterprise Stewardship (NA-53), Ahmad Al-Daouk, presented the award on November 8 in Los Alamos.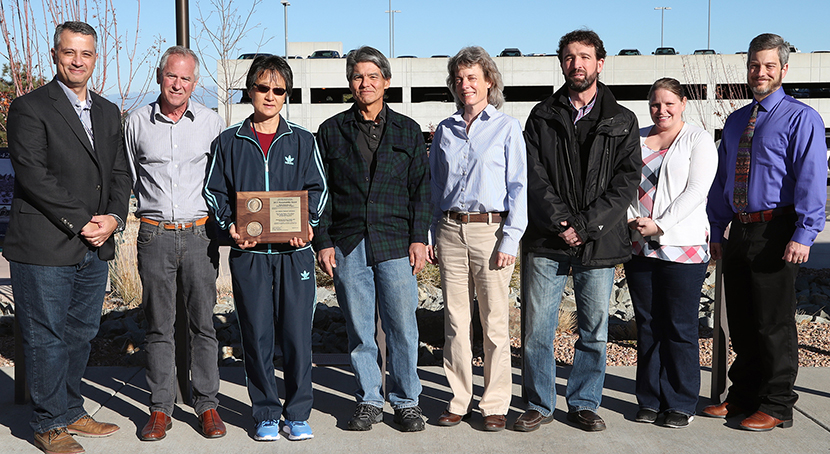
Left to right: Ahmad Al-Daouk (NA-53), Chris Leibman (MPA-11), Ning Xu (C-AAC), Tom Yoshida (C-PCS), Rebecca Chamberlin(C-AAC), Jose-Maria Sansinena (C-PCS), Lisa Meyers(C-AAC), Wayne Evelo (NA-533).
The team demonstrated that ammonium bifluoride could recovering microgram quantities of uranium oxides (U3O8 and UO2) from filter swipe media. They obtained uranium recoveries of 100% for U3O8 and 90% for UO2. Unlike conventional mineral acids, which cannot be transported by air cargo, solid ammonium bifluoride and dilute solutions have minimal restrictions on their transport and can be used safely in the field.
Uranium (U) oxides are commonly present in nuclear fuel processing plants and nuclear research facilities. Uranium oxide particles collected on the swipe medium are typically dissolved with strong corrosive inorganic acids such as concentrated nitric, hydrofluoric, or hydrochloric acids prior to the analysis for uranium isotopic compositions. Such corrosive chemicals are difficult to use in the field because their transportation is highly regulated. Ammonium bifluoride finds use in consumer products such as glass etchants used by hobbyists, and polish for aluminum wheel covers on cars. Due to its less hazardous chemical properties, ammonium bifluoride has often been used as a replacement for hydrofluoric acid in industrial application.
Reference: “Dissolution of Uranium Oxides from Simulated Environmental Swipes using Ammonium Bifluoride,” Journal of Radioanalytical and Nuclear Chemistry 310, 817 (2016); doi: 10.1007/s10967-016-4823-4. Authors: Lisa A. Meyers (C-AAC), Thomas M. Yoshida (C-CDE), Rebecca M. Chamberlin and Ning Xu (C-AAC).
The works supports the Laboratory’s Nuclear Deterrence and Global Security mission areas and the Materials for the Future science pillar. Technical contact: Ning Xu
Bioscience
Jeanne Fair edits e-book about biothreats and how to reduce them
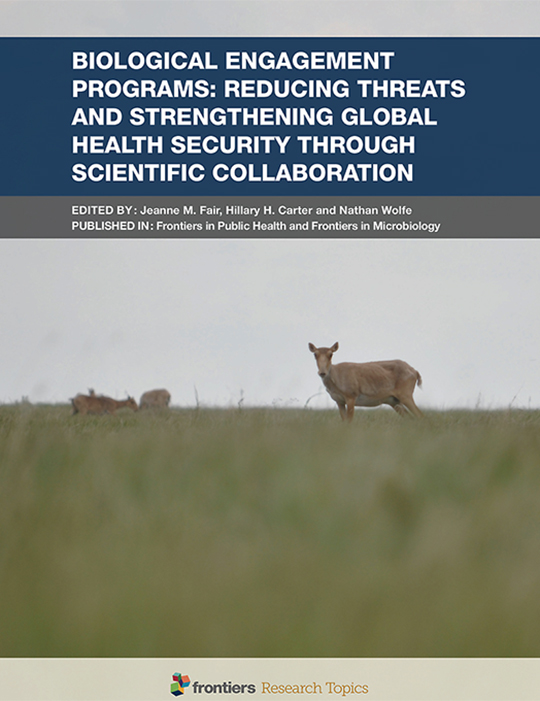
Cover of the book Biological Engagement Programs: Reducing Threats and Strengthening Global Health Security Through Scientific Collaboration.
Los Alamos scientist and Biosecurity and Public Health (B-10) Deputy Group Leader Jeanne Fair is editor-in-chief of a newly released e-book about biothreats and how to reduce them. Titled “Biological Engagement Programs: Reducing Threats and Strengthening Global Health Security Through Scientific Collaboration,” Frontiers published the free e-book online. Fair authored three chapter papers including the opening editorial chapter, and other Los Alamos researchers [Helen H. Cui, Tracy Erkkila, Patrick S. G. Chain (B-10), and Momchilo Vuyisich (formerly B-10)] also wrote chapters.
In her editorial opening chapter, Fair stated that early detection remains one of the best ways to prevent small outbreaks from becoming epidemics and pandemics. Accurate diagnosis, detection, and reporting of diseases are important components of mitigating outbreaks, and biosurveillance remains the top tool in our toolbox. While vaccines have been important for controlling more common infectious virus diseases, they are not as feasible for less common diseases, emerging pathogens, and rapidly evolving microbes. Biosurveillance is critical throughout the world due to globalization and increased travel, emigration, and migration.
Building up the capabilities and capacities for biosurveillance is a global challenge. Cooperative biological engagements help address biosurveillance and biosafety gaps in capabilities and reduce threats worldwide, by strengthening biosurveillance globally in a number of ways. The first is in assisting countries and regions to increase their technical expertise for detecting, diagnosing, and reporting on rapidly changing and emerging infectious diseases. Second, cooperation can help strengthen the biosafety and biosecurity of laboratories around the world. Third, biosurveillance can be strengthened by understanding the best strategies for biosurveillance planning, and the potential epidemiology of a disease system within a region. Collaborative research helps scientists understand a disease system in the environment and devise the most effective strategy for detecting outbreaks.
Most pathogens continually circulate in animal species, enabling a constant interplay between agricultural animals, wildlife, the environment, and humans. Host range and host heterogeneity are important aspects of a disease system, as is identifying dead end hosts, regular host, and potential “super-spreaders.” Rates of evolution of phenotypic traits in species vary widely in a continuum of slow to rapid evolution. Species may adapt to environmental changes differently. International cooperation will continue to be important as vectors, hosts, and pathogens shift their ranges and seasonality.
Selection pressures may also force rapid evolution in species with short generation times, such as microbes. Antimicrobial resistance (AMR) is an example of rapid evolution in response to selection pressures, primarily in response to antimicrobial drugs. AMR is now considered a major global threat to public health because it occurs everywhere in the world, compromising the ability to treat infectious diseases with life-saving drugs of the past such as penicillin. Because antimicrobial selection pressures may vary between country and region, international collaboration is required to tackle the challenge of increasing antimicrobial-resistant pathogens.
In other sections of the book, the Los Alamos authors report that the Laboratory has supported Cooperative Threat Reduction programs for many years, primarily by helping partner countries enhance biosurveillance systems with genomic sequencing to detect pathogens of security concern. This practice is an example of biological engagement, wherein a set of projects or activities between partner countries strengthen global health security to achieve mutually beneficial outcomes. Biological engagement programs reduce the threat of infectious disease with a focus on pathogens of security concern, such as those pathogens identified by the U.S.
Government as Biological Select Agent and Toxins. These programs seek to develop technical or scientific relationships between countries to combat infectious diseases both in humans and animals. Work on laboratory biorisk management, diagnostics, pathogen detection, biosurveillance and countermeasure development for infectious diseases fosters deep relationships between countries. Biological engagement programs are also designed to address dual-use issues in pathogen research by promoting responsible science methodologies and cultures. Scientific collaboration is a core mechanism for engagement programs designed to strengthen global health security, including prevention of avoidable epidemics; detection of threats as early as possible; and rapid and effective outbreak response.
Through biological engagement and the Cooperative Threat Reduction program Los Alamos scientists have contributed to the establishment of genome centers with public health and research institutions the Republic of Georgia, Kingdom of Jordan, Uganda, and Gabon, as well as broader collaborations in genomics applications with research institutions in many other countries. These contributions included everything from consultation on the physical design of the genome centers and the equipment needed to training international scientists how to conduct the sequencing and analyze the results.
Reference: Editors: Jeanne. M. Fair (B-10), Hillary H. Carter (U.S. Department of State), Nathan Wolfe (Metabiota). (2017). Biological Engagement Programs: Reducing Threats and Strengthening Global Health Security Through Scientific Collaboration. Lausanne: Frontiers Media. doi: 10.3389/978-2-88945-273-6.
The US Defense Threat Reduction (DTRA) Agency Cooperative Biological Engagement Program and the US Department of State Biosecurity Engagement Program funded the work, which supports the Lab’s Global Security mission area and the Science of Signatures science pillar through biosurveillance for pathogens. Technical contact: Jeanne Fair
Chemistry
Using artificial neural networks to design better biofuels
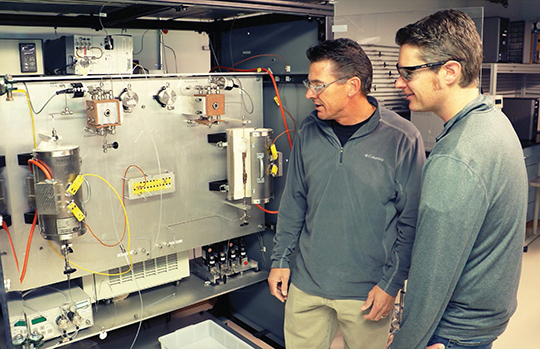
Troy Semelsberger (left) and Andrew Sutton stand alongside the continuous flow reactor that their team developed. The high-throughput environment could improve biofuels production by enabling more efficient chemical conversion of biomass into hydrocarbon fuels.
Los Alamos researchers have developed a novel method to predict the quality of new biomass-derived fuels and fuel mixes. Their approach uses artificial neural networks (ANNs) to estimate the combustion characteristics of fuel constituents and new fuel molecules without having to produce large quantities for physical testing, making it a potentially a powerful tool for designing renewable transportation fuels.
The majority of advanced biofuels research is devoted to exploring efficient ways of converting nonfood biomass into fuels that are compatible with the existing transportation infrastructure, engine technologies, and government regulations. The pursuit of low-cost next-generation biofuels has prompted interest in synthesis via novel chemical pathways, which often yield hydrocarbons and oxygenated organic compounds that are not found in current fuel supplies and whose physical and chemical properties have not been measured or published, though their molecular structures are known. Physical testing of potential fuel compounds can be expensive and time consuming, and if the chemicals are not available commercially, considerable time and effort may be needed to produce testable quantities. For example, ignition quality testing for fuels typically requires between 100 mL and 500 mL, depending on fuel type. Producing these quantities for testing purposes can be impractical, requiring assumptions as to what constitutes a good fuel blendstock molecule based on a qualitative assessment of chemical structure.
The Los Alamos team aimed to develop a reliable method for estimating the physical and chemical properties of fuel components from molecular structure, which in turn distinguishes promising fuels from mediocre fuels. Their quantitative approach is based on the relationship of molecular structure to molecular properties, which means determining the connection between the molecular structure and how efficiently it burns. This quantitative structure−property relationship, or QSPR, is a standard means of determining thermodynamic and transport properties of molecules, but it is only now being applied to fuels.
Determining QSPR is a complex process. Therefore, the team applied an analysis technique called “artificial neural networks,” or ANNs. These ANNs are established computational tools for solving complex problems. By mimicking biological neural networks, the ANN algorithms learn and progressively improve outputs such as predictions about the behavior of fuel constituents or blends. The ANN the Los Alamos team developed accounts for the complex, nonlinear interactions among functional groups better than other methods, and it is applicable to a broad range of hydrocarbons and oxygenated organic compounds. Functional groups are sets of either atoms or bonds between atoms that determine how molecules behave during chemical reactions. The researchers used ANNs to predict the contributions of various functional groups to the overall characteristics of fuels and fuel blends.
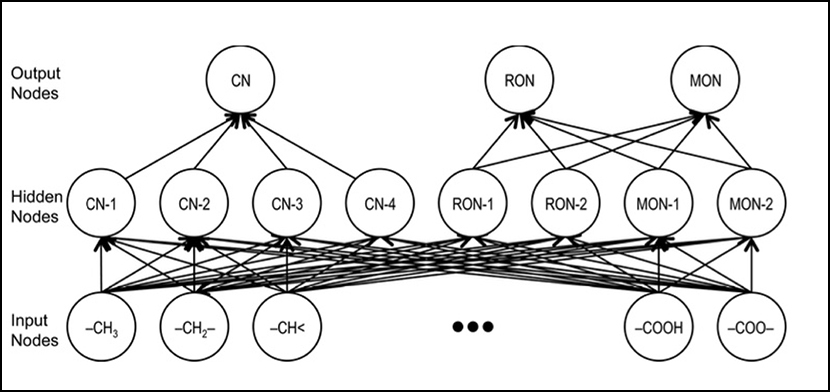
Architecture of the ANN for predicting centane number (CN), research octane number (RON), and motor octane number (MON) from the group structure of a fuel molecule. Centane number is a measure of the suitability of a compound as a diesel fuel.
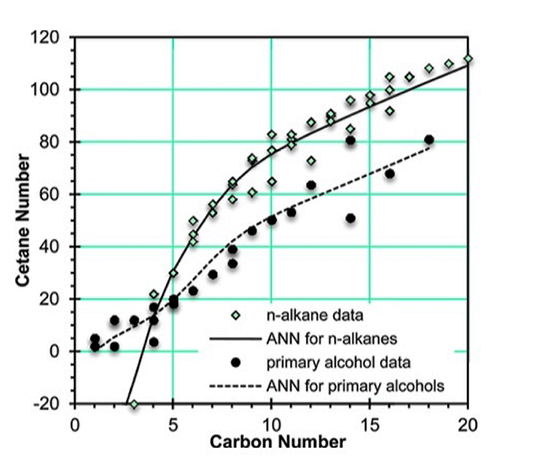
This graph shows ANN-based group contribution method results for the centane number (CN) of n- alkanes and primary alcohols of n-alkanes. The predicted results and the experimental results agree well. “Centane number” is a measure of the suitability of a compound as a diesel fuel.
The journal Industrial and Engineering Chemical Research published the research. The method could have significant impact on the design of the next generation of biofuels. The team has offered their ANN tool to other researchers via an easy-to-use, Excel-based implementation that will predict how functional groups contribute to overall fuel characteristics.
Reference: “Artificial Neural Network Based Group Contribution Method for Estimating Cetane and Octane Numbers of Hydrocarbons and Oxygenated Organic Compounds.” Industrial and Engineering Chemistry Research 56, 12236 (2017); doi: 10.1021/acs.iecr.7b02753. Authors: William L. Kubic, Jr., (Process Modeling and Analysis, AET-2), Rhodri W. Jenkins, Cameron M. Moore, and Andrew D. Sutton (Inorganic, Isotope and Actinide Chemistry, C-IIAC) and Troy A. Semelsberger (Materials Synthesis and Integrated Devices, MPA-11).
The Laboratory Directed Research and Development (LDRD) program funded the work, which supports the Lab’s Energy Security mission area and the Materials for the Future science pillar through the capability to design better biofuels. Technical contact: Andrew Sutton
Earth and Environmental Sciences
Predicting first-passage times in sparse discrete fracture networks
Predicting how long it takes for a chemical to be transported through sparse fracture networks is a common and critical challenge in many subsurface applications including the detection of clandestine low-level nuclear tests, hydrocarbon extraction, aquifer storage and management, environmental restoration of contaminated fractured media, and geological carbon dioxide (CO2) sequestration. Modern subsurface flow and transport models can provide estimates for these transit times, commonly referred to as first passage times, but are hindered by the high computational expense associated with explicitly representing complex fracture networks and ensemble analysis required to quantify uncertainty.
Los Alamos researchers have designed a methodology to identify sub-regions in fracture networks where the fastest transport occurs. The method leverages two Laboratory-developed software suites, NetworkX and dfnWorks, to provide accurate estimates of first passage times with an order of magnitude reduction in computational cost. The cornerstone of the method is combining the topology discrete fracture network with boundary conditions of the simulation to derive a graph representation where nodes in the graph correspond to fractures in the network and nodes are connected if two fractures intersect. This graph is amenable to network analysis tools that allowed for efficient query of the network structure. The journal Physical Review E published the method.
The team demonstrated that identifying the subgraphs corresponding to the shortest paths between the inflow to outflow boundaries can be used to accurately predict where the fastest particles pass through the fracture networks. The Figure provides an overview of their new method. Subfigure (a) shows a discrete fracture network (DFN) generated using the dfnWorks software suite. Subfigure (c) is a graph representation of that network where fractures are represented as nodes and edges exists between nodes if the corresponding fracture intersect. Additional nodes are added to incorporate the inflow (red) and outflow (blue) boundary conditions. Subfigure (d) shows the subgraph corresponding to 10 shortest paths from inflow to outflow boundaries, identified using NetworkX, and (b) is the corresponding sub-network within the DFN. Subfigure (e) compares the first arrival times of particles in the full network and subnetworks corresponding to the 10 shortest paths in a set of 100 networks. The results are in good agreement demonstrating the accuracy of the method. The required CPU times are presented in subfigure (f) and highlight that simulating flow and transport in the sub-network is an order of magnitude less expensive that the full network demonstrating the method’s efficiency.
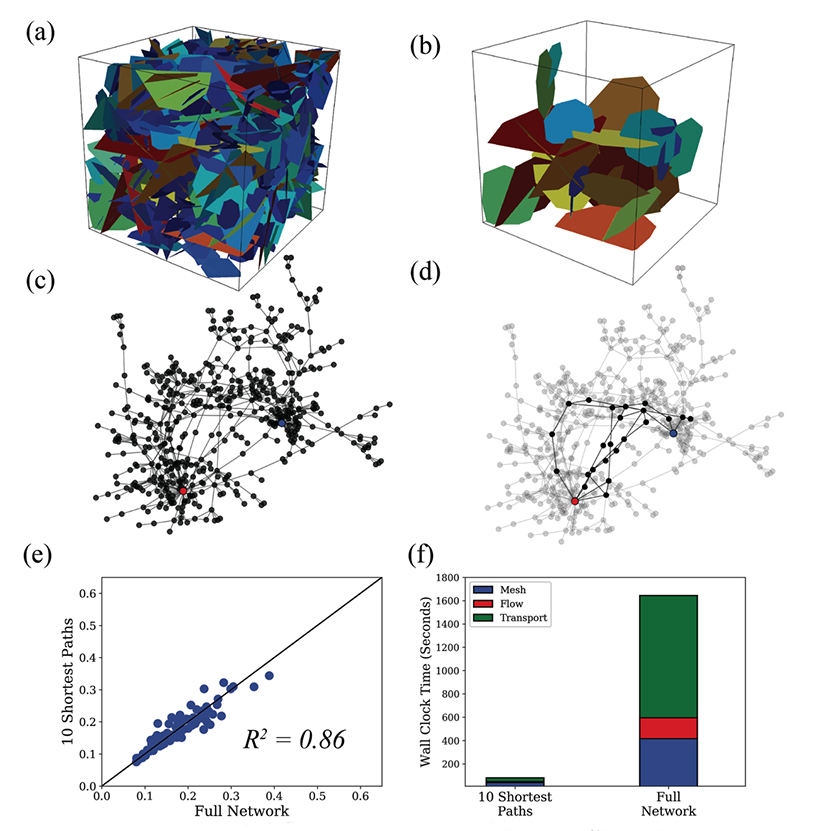
Graph-based methods identify sub-regions in fracture networks where the fastest transport occurs. (a) Full network, (b) subnetwork corresponding to the 10 shortest paths between flow boundaries, (c) graph representation of (a), (d) 10 shortest paths between flow boundaries in the graph, (e) comparison of first passage times in the full network and subnetwork based on the 10 shortest paths, (f) comparison of computational times.
Reference: “Predictions of First Passage Times in Sparse Discrete Fracture Networks using Graph-based Reductions,” Physical Review E 96, no. 1 (2017): 013304; doi: 10.1103/PhysRevE.96.013304. Authors: Jeffrey D. Hyman and Hari Viswanathan (Computational Earth Science EES-16), Aric Hagberg and Gowri Srinivasan (Applied Mathematics and Plasma Physics T-5), and Jamal Mohd-Yusof (Applied Computer Science, CCS-7).
The Laboratory Directed Research and Development (LDRD) program and a Laboratory Director’s Postdoctoral Fellowship supported this research through funding provided to “Advancing Predictive Capability for Brittle Failure Using Dynamic Graphs” (Principal Investigator, Hari Srinivasan). Hyman received partial support through a Laboratory Director’s Postdoctoral Fellowship. The work supports the Lab’s Global Security Nuclear Non-proliferation and Energy Security mission area areas, and the Information, Science, and Technology science pillar through prediction of transit times through sparse fracture networks. Technical contact: Jeffrey Hyman
Explosive Science and Shock Physics
X-ray laser experiments show that scientists have overestimated meteor sizes
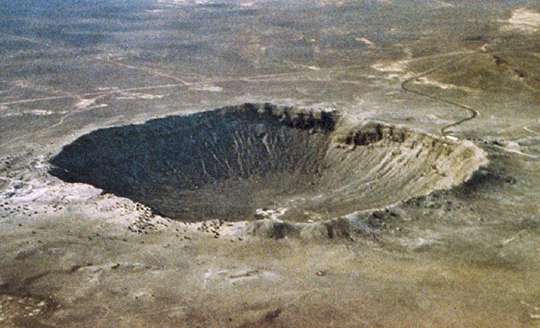
Meteor Crater, AZ where shock metamorphosed minerals can be found, including shocked silicate glass – as was made in the Matter in Extreme Conditions (MEC) target chamber at the Stanford Linear Accelerator facility (SLAC) in California. Photo credit: NASA
During a collision between two bodies, e.g., the impact of a meteor with the Earth, rocks are suddenly subjected to very high pressures and temperatures resulting in shock metamorphism. Knowledge of formation processes experienced by shocked materials is based on ex situ analyses of recovered samples and theoretical analyses. Understanding how rock-forming minerals transform under shock loading is critical for modeling collisions between planetary bodies, interpreting the significance of shock features in minerals and for using them as diagnostic indicators of impact conditions, such as shock pressure. Los Alamos scientists and collaborators have used the high-brightness, short-pulse Linac Coherent Light Source X-ray free electron laser at the SLAC National Accelerator Laboratory to simulate what happens when a meteor hits the Earth’s crust, generating shocked glass. Nature Communications published the research.
Scientists have long known that impacts from meteors can convert silicates into an amorphous phase known as diaplectic, or shocked, glass. The question is how this shocked glass forms – does the rock have to become molten first or can a glass result from the compression release process? New findings indicate that a shocked glass can form on release without melting.
In the past, scientists have tried to estimate the amount of pressure needed to cause this transformation by examining debris from meteor impacts and squeezing mineral samples in pressure cells in the lab, but they were unable to observe the process as it unfolded. The new study showcases the first-ever shock-release amorphization of a silicate step-by-step via femtosecond (10-15 second) X-ray diffraction (XRD). In situ XRD shows that the peak pressure needed to form shocked glass is 25% lower than previously thought. This could lead to an inflation in assumed impactor size. These results, made using an intense laser beam to create a shock wave, lead to a greater understanding of important problems in shock physics and materials science.
The Matter in Extreme Conditions facility combines SLAC’s Linac Coherent Light Source with high power optical laser beams, and a suite of dedicated diagnostics tailored for warm dense matter physics, high pressure studies, shock physics, and high energy density physics.
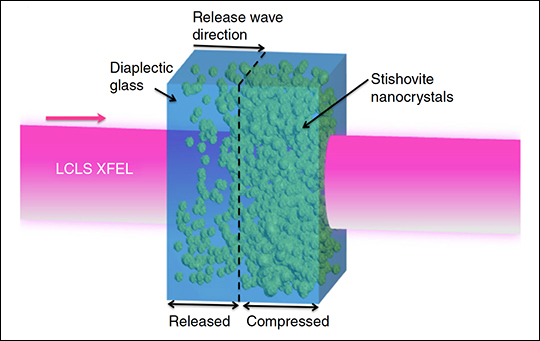
Schematic showing the formation of shocked glass in the wake of a release wave as tiny crystallites of the mineral stishovite (green sphere-like features) dissolve away due to high pressure phase metastability.
In situ XRD, such as available at the Linac Coherent Light Source and planned at the center of MaRIE (Matter-Radiation Interactions in Extremes), LANL’s proposed experimental facility for time-dependent materials science at the mesoscale, provides a unique tool to study materials under extreme conditions. This is the first demonstration of high-pressure metastability mapping with ultrafast X-ray diffraction. These techniques advance the understanding of non-equilibrium phase transformation pathways, which could lead to new materials design with improved functionality. MaRIE, and continued dynamic materials research in general, would take this research further by allowing structural determination of non-crystalline materials and by performing the time-dependent measurements on a single shock event. Additional dynamic drivers could enable longer time measurements to investigate metastable phase boundaries.
Reference: “Time-resolved Diffraction of Shock-released SiO2 and Diaplectic Glass Formation,” Nature Communications 8, 1481 (2017); doi: 10.1038/s41467-017-01791-y. Authors: Arianna Gleason (Shock and Detonation Physics, M-9 and SLAC National Accelerator Laboratory), Cindy Bolme (M-9), Richard Sandberg (Center for Integrated Nanotechnologies, MPA-CINT), H. J. Lee, B. Nagler, and E. Galtier (Linac Coherent Light Source, SLAC), R. G. Kraus (Lawrence Livermore National Laboratory; W. Yang (Center for High Pressure Science and Technology Advanced Research, China and Carnegie Institution of Washington); F. Langenhorst (University of Jena, Germany); and W. L. Mao (SLAC National Accelerator Laboratory and Stanford University).
The Laboratory Directed Research and Development (LDRD) program sponsored the Los Alamos work, and a Reines Distinguished Postdoctoral Fellowship funded Gleason. The research supports the Lab’s Nuclear Deterrence mission area and the Materials for the Future science pillar by demonstrating a strategy and methodology to extract phase transition kinetics using time-resolved x-ray diffraction data. Technical contact: Arianna GleasonIntelligence and Space Research
Discovery of boron on Mars sheds light on its past environment
The recent discovery of boron on Mars has given scientists a rare glimpse into what may have been a rather mild and wet environment billions of years ago. The presence of boron is another line of evidence that water once existed on Mars in environmental conditions ripe for prebiotic chemistry. Borates are one possible bridge from simple organic molecules to ribonucleic acid (RNA), one of the building blocks of life. Finding boron on Mars further opens the possibility that life could have once arisen on the planet. Some scientists believe that without RNA there would have been no formation of life on early Earth. Thus, the presence of boron on Mars provides researchers with new evidence that if organics were present, these chemical reactions could have occurred.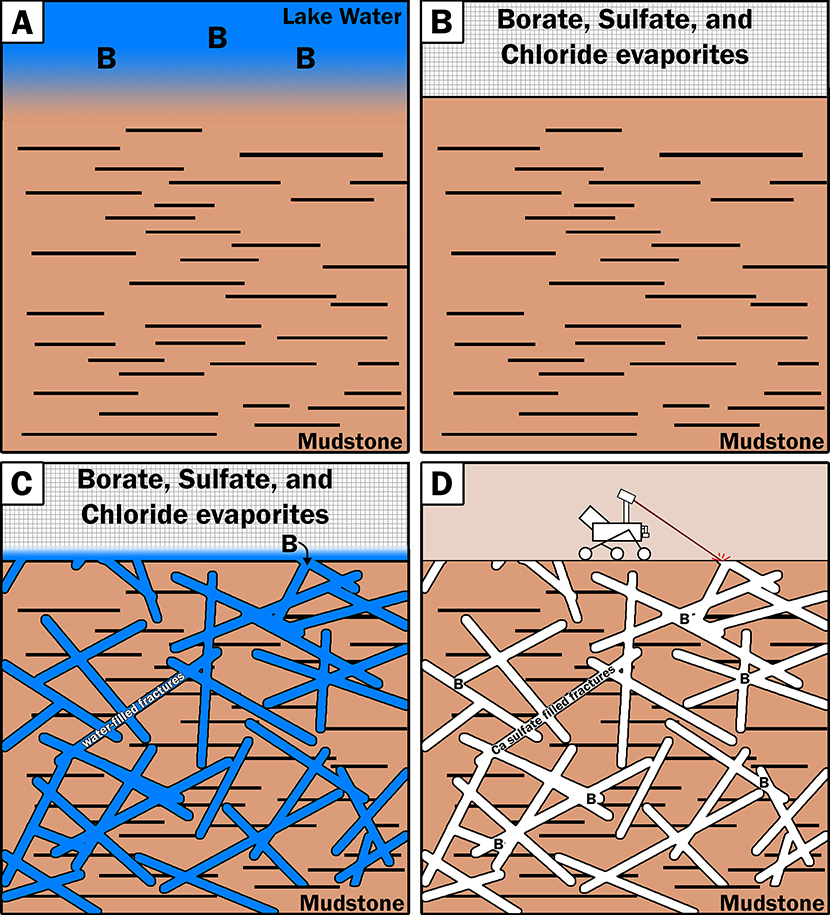
The hypothesis for how boron ended up in calcium sulfate veins is a multi-step process. A) Boron was dissolved in the lake as lakebed mudstone deposits formed. B) When the lake evaporated, salts containing boron, sulfur, and chlorine salts were deposited on top of lakebed deposits. C) Later groundwater formed fractures in the impermeable lakebed deposits, and some of the salt deposits were re-dissolved by this groundwater. This caused some of the boron to be recirculated along with the groundwater. D) After billions of years of erosion, the current surface of the lakebed mudstone and veins were exposed. The rover was then able to analyze them with ChemCam.
The report of boron on Mars by a team of researchers lead by Los Alamos postdoc Patrick Gasda (Intelligence and Space Research, ISR-2), published in Geophysical Research Letters, represents the first time this chemical element has been observed by any instrument on Mars. Although Mars is cold and arid on its surface today, scientists have hypothesized that water was present on the Martian surface in the planet’s very distant past i.e. 2-4 billion years ago. Boron on Mars was discovered in material that likely formed in groundwater that ranged between 0-60 degrees Celsius (32-140 degrees Fahrenheit). The presence of boron in the groundwater is a good indication that the water was neutral-to-alkaline pH, and potentially habitable for microbial life.
Researchers have hypothesized that boron was dissolved in Martian groundwater, which circulated underground for perhaps hundreds of millions of years, as depicted in the Figure. If organics were also present during this time, scientists suggest that this groundwater would be a potential place for prebiotic chemical reactions to occur. Prebiotic reactions are chemical reactions needed to form the necessary components for the origin of life. One such molecule is RNA. Researchers have hypothesized that the formation of RNA on early Earth was a key step before the formation of cellular life. Boron could have played a key role in the formation of RNA by reacting with ribose (the “R” in RNA) and preventing its decomposition in water. The discovery of boron in an ancient groundwater system opens up the possibility that prebiotic reactions between boron and ribose could have occurred, and that life could have independently arisen on Mars.
In 2012 two events occurred that began this journey to discovery. Scientists at the University of Hawaii were studying a number of Martian meteorites found in Antarctica. During the characterization of these meteorites, researchers found traces of boron along with other minerals found in water. This inspired Patrick Gasda, working towards his Ph.D. in geology at the University of Hawaii at that time, to pursue research in boron on Mars. Also in the summer of 2012, NASA’s Curiosity Rover arrived on the Martian surface. Equipped with an instrument suite that includes a laser-induced breakdown spectroscopy (LIBS) instrument and a remote micro-imager (RMI), Curiosity has the ability to probe small targets and collect geologic context and color images.
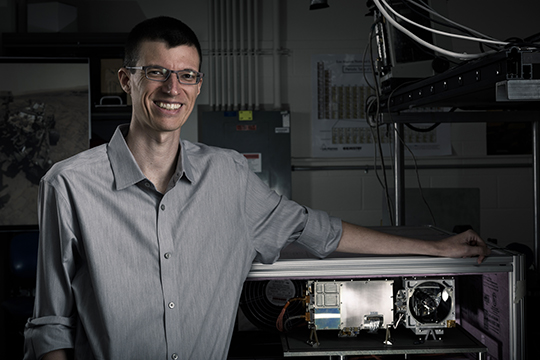
Patrick Gasda with the ChemCam engineering unit at TA-35.
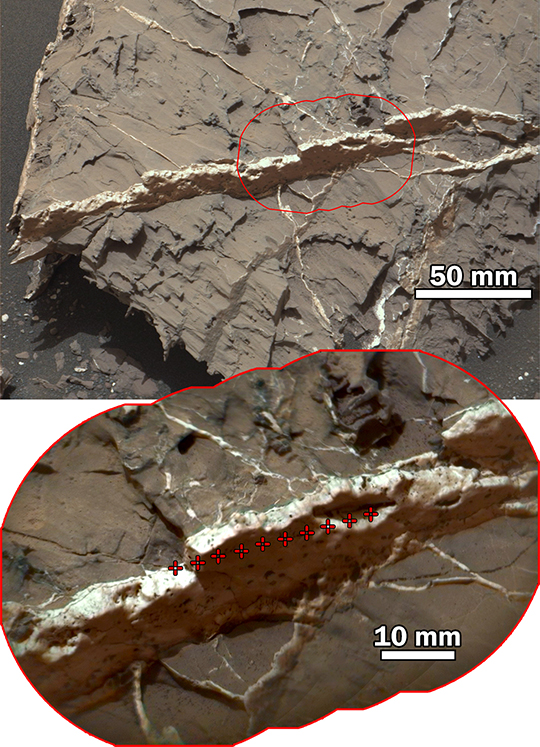
The top panel shows the Mastcam image of the target "Catabola," analyzed by ChemCam on Sol (mission day) 1443, which had significant quantities (tens of parts per million) of boron. The bottom panel is the ChemCam remote micro image of the target with crosshairs showing each place ChemCam hit the target with the laser. The light-toned material is calcium sulfate protruding above tan-colored lakebed rocks.
After completing his Ph.D., Gasda joined the Los Alamos team working with the Mars Rover seeking to find the boron signal in the ChemCam data. Gasda and undergraduate summer student Ethan Haldeman (Ursinus College), began analyzing boron-containing terrestrial samples using a copy of the ChemCam instrument located in a laboratory at TA-35.
The lab instrument targets rock samples in a small chamber that replicates the Martian atmospheric pressure and composition. Gasda and Haldeman mathematically modeled the boron signal observed in the laboratory data, and then used this model to find the same signature of boron in ChemCam data from Mars. They delved into four years of ChemCam data, hundreds of thousands of spectra, and found a few percent that contained the boron signal. The boron was found in calcium sulfate filled “veins” which stood out as white material among lakebed rocks in Gale Crater.
The Los Alamos scientists have concluded that boron was present in a long-lived groundwater system, suggesting that important prebiotic chemical reactions could have occurred in the groundwater if organics were also present. As a result, an additional piece to the puzzle has now been added and evidence suggests that the basic foundation of mild temperatures, water, and boron existed together at one time on Mars, making for a habitable environment.
Reference: “In situ detection of boron by ChemCam on Mars,” Geophysical Research Letters 44, 8739 (2017); doi:10.1002/2017GL074480.
Authors: Patrick Gasda, Roger Wiens, Nina Lanza, and Madeleine Bodine (Intelligence and Space Research, ISR-2); Samuel Clegg and Rhonda McInroy (Physical Chemistry and Applied Spectroscopy, C-PCS); and Dorothea Delapp (Space Data Science and Systems, ISR-3) of Los Alamos National Laboratory; Ethan Haldeman and Veronica Sanford (Ursinus College); William Rapin (California Institute of Technology): John Bridges (University of Leicester, UK); Thomas Bristow (NASA Ames); Susanne Schwenzer (Open University, UK); Benton Clark (Space Science Institute); Kenneth Herkenhoff (U.S. Geological Survey, USGS); Jens Frydenvang (Natural History Museum of Denmark and University of Copenhagen); and Sylvestre Maurice (Centre National d’Etudes Spatiales, CNES and the Institut de Recherche en Astrophysique et Planétologie, IRAP).
ChemCam was developed by Los Alamos National Laboratory scientists through funding from NASA’s Mars Exploration Program, and in collaboration with CNES, the French space agency.
This research supports the Laboratory’s Global Security mission area and the Science of Signatures science pillar through the development of methods for rapid chemical analysis in extreme environments and remote sensing for situational awareness in space. Technical contact: Patrick Gasda
Materials Physics and Applications
Atomic-level observations reveal how low-cost fuel cell catalysts work
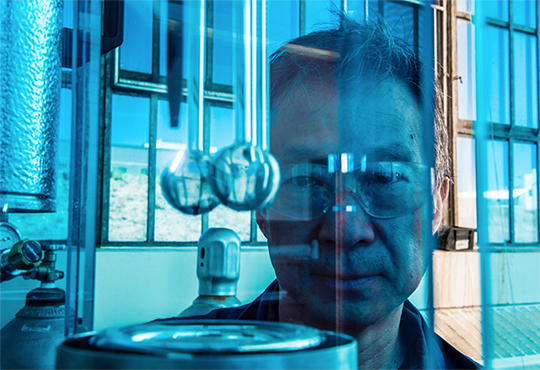
Researcher Hoon Chung stands behind a Quantachrome Instruments gas sorption analyzer, which measures the surface area and pore-size distribution in fuel cell electrocatalysts.
The widespread integration of polymer electrolyte fuel cells into vehicles will require substantial reductions in overall stack cost. To reduce the cost of next-generation fuel cells, researchers have been developing alternatives to the prohibitively expensive and scarce platinum and platinum-group metal (PGM) catalysts currently used in fuel cell electrodes. A study by Los Alamos researchers and collaborators at Oak Ridge National Laboratory accelerates those efforts by determining efficient, new, more abundant materials and discovering how they function at an atomic level. The journal Science published the research.
The Los Alamos-led team built on their previous studies to synthesize oxygen reduction reaction (ORR) catalysts made of low-cost platinum alternatives that perform comparably to the standard PGM fuel cell catalyst used in vehicle applications. The new material used in this study is an iron-nitrogen-carbon (Fe-N-C) fuel cell cathode electrocatalyst, synthesized with two nitrogen precursors. The hierarchical porosity of the resulting material exposes much of the catalytically active sites to oxygen.
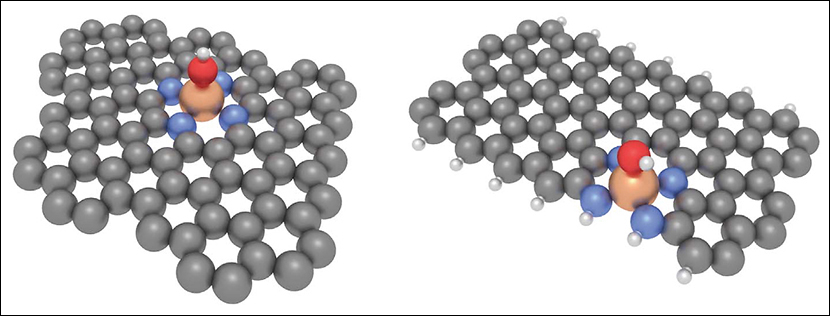
Views from a tilted perspective show the model structures used in theoretical studies with spontaneously formed OH ligand. The left image shows the bulk-hosted FeN4; the right image shows zigzag edge-hosted FeN4 structures with OH ligands. Carbon, gray; iron, bronze; hydrogen, white; nitrogen, blue; oxygen, red.
Scientists used sophisticated microscopy at Oak Ridge National Laboratory to make the first direct observations of the single-atom active sites in the novel material where catalysis takes place. This information provides unique insights into the ORR mechanism at the surface of Fe-N-C catalysts.
Fuel cell test-stand performance demonstrates that the material’s fuel-cell performance approaches that of platinum catalysts. Computer modeling conducted at Los Alamos predicted the high activity of Fe-N-C catalysts, the FeN4 active-site structure, and the possible reaction pathway. Quantum chemistry calculations indicated that: 1) ORR on the FeN4 sites follow different ORR reaction pathways, depending on whether they are hosted in the bulk or at the edge of the graphene, and 2) that the edge-hosted sites, when spontaneously ligated by OH in the fuel cell environment, lead to highly ORR-active structures. These results confirm that the sites observed by microscopy are likely responsible for the high ORR activity of the synthesized catalyst. This information provides insight into the development of reliable, affordable catalysts to enable large-scale commercialization of fuel cell-powered vehicles.
Reference: “Direct Atomic-level Insight into the Active Sites of a High-performance PGM-free ORR Catalyst,” Science 357, 479 (2017); doi: 10.1126/science.aan2255. Authors: Hoon T. Chung, and Piotr Zelenay (Materials Synthesis and Integrated Devices, MPA-11); Drew Higgins (formerly LANL, now at Stanford University); David A. Cullen, Brian T. Sneed, and Karren L. More (Oak Ridge National Laboratory); and Edward F. Holby (Sigma Division, Sigma-DO).
Patent: U.S. patent number US20160351915 A9, “Non-precious Metal Catalysts,” covers the binary nitrogen precursors PGM-free catalyst synthesis method. Inventors: Hoon T. Chung and Piotr Zelenay (MPA-11).
The DOE Office of Energy Efficiency and Renewable Energy’s Fuel Cell Technologies Office funded the experimental work at Los Alamos, and the Laboratory’s Institutional Computing program provided the computational resources. The research into fuel cells expands options for energy production supports the Laboratory’s Energy Security mission area and the Materials for the Future science pillar. Technical contact: Piotr Zelenay
Nuclear Engineering and Nonproliferation
Advanced formalism enhances neutron counting capability for nuclear safeguards
The science of international nuclear safeguards uses neutron multiplicity counting for the identification, verification, and assay of special nuclear materials. Neutron multiplicity counting relies on the temporally correlated analysis of detected-neutron pulse times (pulse train) that provides a unique signature of spontaneously fissioning materials. This temporally correlated nature can be described by a vector of counting rates: Singles (S), correlated pairs (Doubles, D) and correlated triplets (Triples, T). The three counting rates are used, in combination with an analytical model known as point-model, to calculate the characteristics of the measured special nuclear material mass of plutonium, its multiplication and contribution of uncorrelated neutrons from (α,n) reactions (alpha particle reacts with a nucleus, followed by the subsequent release of a neutron). All three quantities are essential components in reliable characterization of special nuclear material through neutron multiplicity counting, which represents a well-established technique for nuclear safeguards and nonproliferation applications.
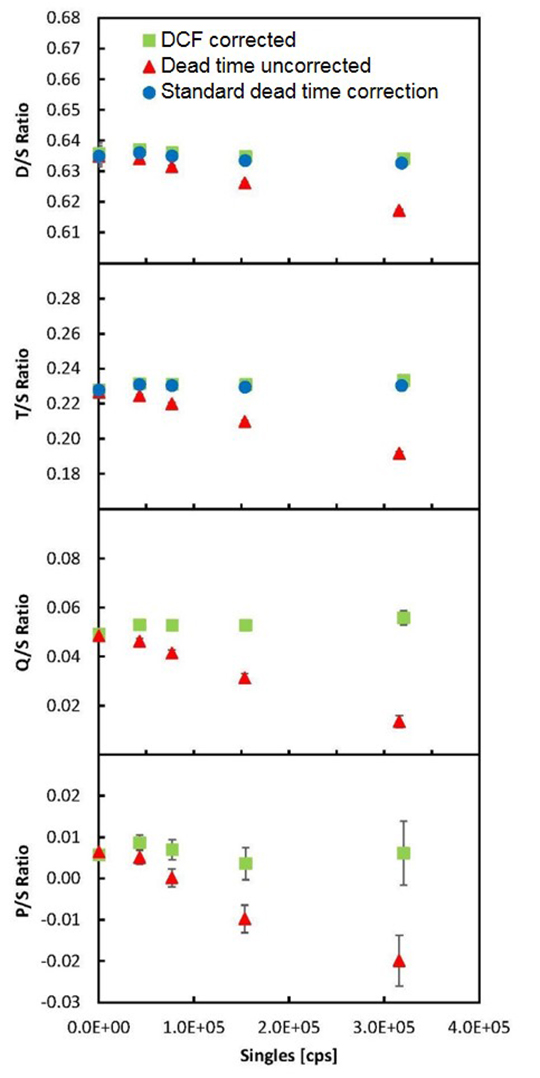
DCF corrected count rate ratios for a set of 252Cf sources measured in a high-efficiency multiplicity counter as a function of Singles rates. The red data points show the results before the DCF dead time correction treatment, and the blue data points validate the new dead time treatment against the currently available standard that is only developed up to Triples.
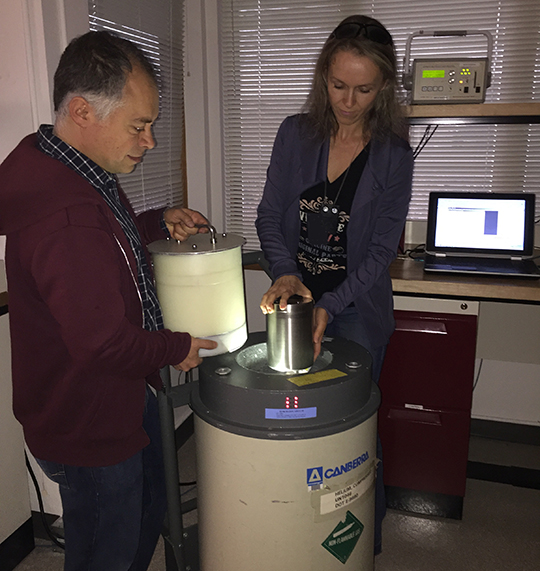
Andrea Favalli and Daniela Henzlova setting up a measurement.
With improved data acquisition techniques and availability of high efficiency neutron multiplicity detectors, the feasibility and implications of extending the neutron multiplicity counting beyond Triples can be assessed. The addition of higher order correlated rates (Quads, Q, and Pents, P) expands experimental information obtained from the special nuclear material measurement and can aid in its improved characterization. Dedicated dead time correction methods are required to be able to use these additional observables.
The Nuclear Engineering and Nonproliferation (NEN-1) group in collaboration with Oak Ridge National Laboratory developed one such method, called the Dytlewski-Croft-Favalli (DCF) dead time correction algorithm. This method extends the multiplicity counting towards higher order correlated rates with the self-consistent treatment of dead time. Dead time is the period after each detected event during which the detector system is not able to record another event. The journal Nuclear Instruments and Methods in Physics Research A published the research.
Researchers incorporated the DCF algorithm into a NEN-1 developed software Multiplicity Advanced AlGorithm Implementation Code (MAGIC) for the purpose of evaluation and future use. The availability of high neutron efficiency detectors and suitable neutron sources in the NEN-1 laboratories enabled an experimental evaluation of the DCF algorithms, which the journal article Nuclear Instruments and Methods in Physics Research A reported. The Figure shows the DCF corrected count rate ratios for a set of 252Cf (californium-252) sources as a function of the Singles rates.
The dead time corrected count rate ratios (DCF corrected) exhibit constant trends, independent of source strength, as expected by theory and revealing a very good performance of the dead time algorithm method developed. The investigators also validated the DCF method in measurements of plutonium oxide samples, obtaining results in good agreement with material declaration. The team is examining further application of this technique to allow for advanced material characterization.
The researchers aim to identify and develop a pathway for the exploration of higher order correlated rates to solve practical nonproliferation and nuclear safeguards measurement problems. The method paves a way for improved characterization of some challenging special nuclear materials that cannot be easily, or with sufficient accuracy, assayed using currently available techniques. The practical benefits to other areas, such as benchmark quality subcriticality experimental work to determine basic nuclear data, remain to be assessed but sit on the cutting edge of research in this area.
References:
“Extension of the Dytlewki-style Dead Time Correction Formalism for Neutron Multiplicity Counting to any Order,” Nuclear Instruments and Methods in Physics Research A 869, 141 (2017); doi: 10.1016/j.nima.2017.06.032. Authors: Stephen Croft (Oak Ridge National Laboratory) and Andrea Favalli (Nuclear Engineering and Nonproliferation, NEN-1).
“Experimental Evaluation of the Extended Dytlewki-style Dead Time Correction Formalism for Neutron Multiplicity Counting,” Nuclear Instruments and Methods in Physics Research A 879, 69 (2018); doi: 10.1016/j.nima.2017.09.025. Authors: M. Lockhart (Texas Tech University, NEN-1 student), D. Henzlova (NEN-1), S. Croft (Oak Ridge National Laboratory), T. Cutler (Advanced Nuclear Technology, NEN-2), A. Favalli (NEN-1), C. McGahee (Pennsylvania State University, NEN-1 student), and R. Parker (NEN-1 retired).
The U.S. DOE, National Nuclear Security Administration (NNSA), Office of Nonproliferation Research and Development (NA-22) sponsored the work, which supports the Lab’s Global Security mission area and the Nuclear and Particle Futures and Science of Signatures science pillars through the ability to characterize special nuclear material. Technical points of contact: Andrea Favalli and Daniela Henzlova
Physics
Neutron capture measurements using DANCE improve cross section calculations
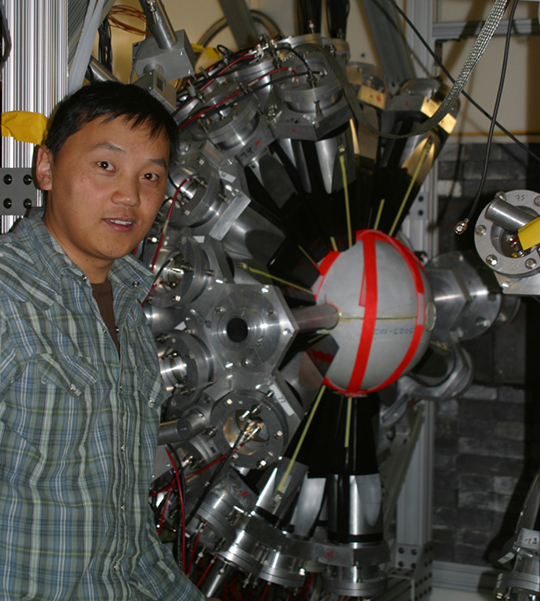
Bayarbadrakh Baramsai [formerly a postdoc in Nuclear and Radiochemistry (C-NR), currently with NASA] with the DANCE detector. The detector has been opened to show individual crystals. This large-area advanced detector, coupled with the intense neutron flux at the Lujan Center, was crucial in making these measurements.
New measurements of neutron capture cross sections (reaction probabilities) and gamma-ray emission spectra completed over several years at Los Alamos Neutron Science Center (LANSCE) have resolved some measurement discrepancies and provided the experimental basis for theoreticians to improve the models used to calculate neutron capture. Researchers used LANSCE’s Detector for Advanced Neutron Capture Experiments (DANCE) to make measurements of uranium (U) isotopes. The improved models may have wide applications in calculating neutron capture for modeling explosion performance, reactor design, test diagnostics, and nuclear forensics. Measurements of cross sections and gamma-ray spectra across the isotope chain supply the needed experimental information and also provide a test of the isotope-dependence of the theoretical models used to describe the reactions. This dependence is important in extending the calculations to unstable isotopes that cannot be easily studied experimentally. Several publications, including Physical Review C, have reported the new measurements.
Many capture cross section measurements have been made, but researchers still see discrepancies between the data, even in uranium-235 (235U), a well-studied nuclide. Measurements are further complicated by the radioactive decay that produces a detector background and limits the amount of material that can be measured. However, the advanced DANCE detector has allowed Los Alamos researchers and their collaborators to measure the 235U capture cross-section and resolve an important discrepancy in the 1 to 5 keV energy region. The team also published measurements on 236U and 238U isotopes and is currently analyzing a measurement of 234U.
Gamma-ray emission spectra provide an important constraint on the calculations. Therefore, the team is also analyzing the gamma-ray spectra from 235U capture to extend the theoretical analysis to isotopes that also have a fission contribution. The ultimate goal is to make accurate capture calculations for nuclei that cannot be measured, such as 237U and 239U. DANCE spectra have confirmed that an additional mode of nuclear excitation – the “scissors mode”, which has been observed in measurements of other nuclear reactions – is required to reproduce the observed gamma-ray spectra. The scissors mode is a bulk oscillation of neutrons and protons in deformed nuclei, where the distribution of protons and neutrons are each pictured as having a football-like shape. In the scissors mode, the proton and neutron footballs oscillate opposite to each other around their common center, much like the opening and closing of scissors.
References:
“Estimation of M1 Scissors Mode Strength for Deformed Nuclei in the Medium to Heavy Mass Region by Statistical Hauser-Feshbach Model Calculations,” M. R. Mumpower, et al., Physical Review C 96, (2017); doi: 10.1103/PhysRevC.96.024612. Authors: M. R. Mumpower and T. Kawano (Nuclear and Particle Physics, Astrophysics and Cosmology, T-2), J. L. Ullmann (LANSCE Weapons Physics, P-27), M. Krtička (Charles University), and T. M. Sprouse (University of Notre Dame).
“Radiative Neutron Capture Cross Section from 236U,” Physical Review C 96, (2017); doi: 10.1103/PhysRevC.96.024619. Authors: B. Baramsai, M. Jandel, T. A. Bredeweg, E. M. Bond, A. R. Roman, G. Rusev, and C. L. Walker, (Nuclear and Radiochemistry, C-NR); A. Couture, S. Mosby, J. M. O’Donnell, and J. L. Ullmann (P-27); and T. Kawano (T-2).
“Constraining the Calculation of 234; 236; 238U (n; γ) Cross-sections with Measurements of the γ-ray Spectra at the DANCE Facility, Physical Review C 96, 024627 (2017); doi: 10.1103/PhysRevC.96.024627. Authors: J. L. Ullmann, M. Jandel, A. Couture, R. C. Haight, and J. M. O’Donnell (P-27); T. Kawano (T-2); B. Baramsai, T. A. Bredeweg, R. S. Rundberg, D. J. Vieira, and J. B. Wilhelmy (C-NR); M. Krtička (Charles University); J. A. Becker, A. Chyzh, and C. Y. Wu (Lawrence Livermore National Laboratory); and G. E. Mitchell (North Carolina State University).
The NNSA Science Campaign 1 (Laboratory program manager Ray Tolar), Campaign 4 (Laboratory program manager Melissa Douglas), the Laboratory Directed Research and Development (LDRD) program, the DOE Office of Science U.S. Nuclear Data Program, and a DOE Early Career Research grant to M. Jandel funded different aspects of the research. The LDRD program originally supported the DANCE detector. A DOE Early Career Research grant to M. Jandel funded the 234U and 236U cross section measurements. NNSA science campaigns sponsored the 238U cross section measurements and the measurements of the gamma-ray spectra. The DOE Office of Science/Nuclear Data funded the calculations. The work supports the Laboratory’s Nuclear Deterrence, Global Security, and Energy Security mission areas and the Applied Nuclear Science and Engineering focus area of the Nuclear and Particle Futures science pillar. Technical contact: John Ullman
Program Leadership
David Andersson named lead of DOE consortium focus area
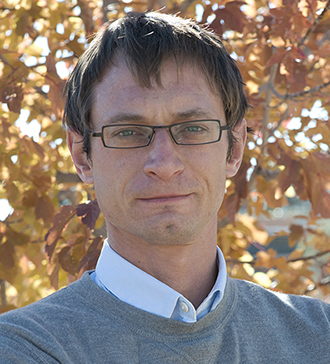
David Andersson
David Andersson (Materials Science in Radiation and Dynamics Extremes, MST-8) has been chosen to lead the Fuels, Materials, and Chemistry focus area of the DOE Consortium for Advanced Simulation of Light Water Reactors (CASL). He succeeds Brian Wirth (University of Tennessee and Oak Ridge National Laboratory).
CASL is a DOE Energy Innovation Hub, research centers that match leading scientists with resources at supercomputing facilities and United States institutions to focus on a single topic for rapid R&D and commercialization of clean energy technologies.
CASL’s goal is to develop modeling and simulation technology by 2020 that predicts the performance of existing operating nuclear reactors. This information could create safer, more efficient, and less costly nuclear power. Los Alamos is a partner in the collaboration, which Oak Ridge National Laboratory leads. CASL has developed VERA (for Virtual Environment for Reactor Applications), a R&D 100 award-winning technology that can simulate nuclear reactor operation down to the characteristics of a single fuel rod.
Research in CASL’s Fuels, Materials, and Chemistry (FMC) focus area guides the development of models and simulation capabilities to tackle core challenges in reactors, including pellet-cladding interaction, fuel rod wear resulting from grid-to-rod fretting, and deposition of corrosive products on cladding. This focus area also addresses ways to assess the implications of loss of coolant accidents and reactivity insertion accidents.
As FMC lead, Andersson will formulate research directions and priorities, coordinate with other CASL focus areas that rely on FMC’s modeling tools, and ensure work is properly executed. Previously, as part of CASL, Andersson helped develop thermodynamic models of Chalk River unidentified deposits (CRUD), which can compromise fuel rods, and thus the performance of nuclear reactors. As deputy FMC lead, he focused on CRUD and the issue of CRUD-induced power shifts and localized corrosion.
Andersson received a Ph.D. in materials science from Sweden’s Royal Institute of Technology. He joined the Lab as a Seaborg Institute Postdoctoral Fellow in 2007, and became a staff member in 2009. Andersson is recognized for his work applying density functional theory calculations and atomistic simulations to multi-scale modeling of actinide and lanthanide oxides. He has performed pioneering, award-winning work on multiscale modeling of nuclear fuels as part of the Nuclear Energy Advanced Modeling and Simulation program, which the DOE Office of Nuclear Technology sponsors. His research supports the Laboratory’s Energy Security mission area and the Nuclear and Particle Futures and Integrating Information, Science and Technology for Prediction science pillars through the goal of developing safe, sustainable nuclear energy. Technical contact: David Andersson
Science on the Roadmap to MaRIE
Dynamic material science community offers recommendations for MaRIE
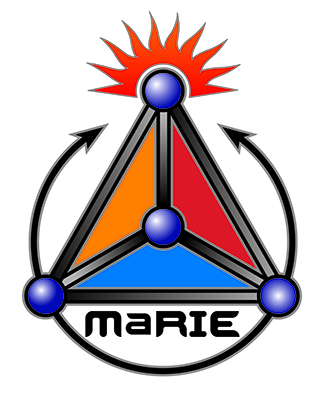
More than 60 experts in dynamic materials science from around the world gathered August 28-29 in Santa Fe to discuss the science requirements and technologies needed for MaRIE (Matter-Radiation Interactions in Extremes), the Laboratory’s proposed experimental facility for studying matter-radiation interactions in extremes. MaRIE’s combination of a unique hard x-ray free electron laser and in situ characterization tools would fulfill a NNSA national security mission need to understand and test how materials structures, defects, and interfaces determine performance of materials in extreme environments.
The participants at the “MaRIE Extreme Environments and Driver Technologies Workshop” considered the technologies, examined possible design issues, and identified critical research and development efforts needed to drive materials samples into the relevant extreme conditions, and thus ensure project success. The workshop will issue a report in the second quarter of FY 2018 to satisfy a Level 2 milestone. That report will provide community recommendations for the path forward both for the facility’s design and for investments at present facilities.
MaRIE would simultaneously characterize materials’ microstructure and response at the mesoscale, filling a gap between the integral scale addressed by hydrotest facilities such as the Los Alamos Dual Axis Hydrodynamic Test Facility and the Nevada National Security Site’s U1A complex, and facilities for materials phenomena at small scale such as Lawrence Livermore National Laboratory’s National Ignition Facility and Sandia National Laboratories’ Z Pulsed Power Facility. Many of the remaining uncertainties in our assessment of materials arise from uncertainties in properties governed at this mesoscale.
Workshop sessions discussed current and planned capabilities at existing facilities, and additional challenges for the MaRIE capability such as those related to higher-energy density materials, plasmas, and hydrodynamic flow. The event featured two sets of breakout sessions. One focused on MaRIE experimental requirements for studies of metal alloys, high explosives and heterogeneous materials, hydrodynamics and turbulent flow, and high energy density physics. The second set of breakouts helped define the technical functional requirements and possible design requirements for MaRIE driver technology, including lasers, pulsed power, and more “classic” technologies such as high explosives and gas and powder gun flyers.
Organizing committee members were Gilbert “Rip” Collins (University of Rochester), John Benage (Sandia National Laboratories), Jon Eggert and Rick Krauss (Lawrence Livermore National Laboratory), and from Los Alamos David Moore (Nuclear Materials Science, MST-16), Cris Barnes (MaRIE), Lucy Maestas (Associate Directorate for Experimental Physical Sciences, ADEPS), and Peggy Vigil (Government Affairs and Protocol, GAP). Representatives from international research institutions, NNSA, DOE national laboratories, academia, and military research facilities attended the event. MaRIE supports the Lab’s Nuclear Deterrence mission area and the Materials for the Future and Science of Signatures science pillars through matter-radiation characterization in extreme environments. Technical contact: Cris Barnes
Theoretical
Emergent Hall physics in 2-D quantum materials combines Hall effects of matter and light
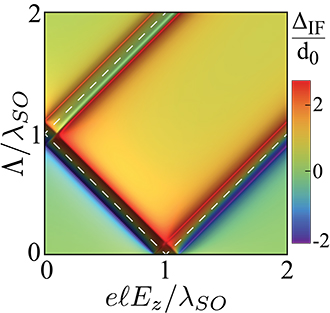
Photonic spin Hall effect phase diagram in two-dimensional topological semiconductors of the graphene family. "Photonic spin Hall effect (\Delta_{IF}/d_0) phase diagram in two-dimensional topological semiconductors of the graphene family versus applied static electric field energy (e\ell E_z) and applied circularly polarized laser coupling energy (\Lambda). Here, lambda_{SO} corresponds to the spin-orbit coupling of the 2-D monolayer.
In a publication in Physical Review Letters, Wilton Kort-Kamp of Physics and Condensed Matter and Complex Systems (T-4) and the Center for Nonlinear Studies (CNLS) investigated the crossroads between topology, phase transitions, spin-orbit interactions, and Dirac physics in two-dimensional materials of the graphene family. The research unveiled the interplay between Hall effects of matter, intrinsic to these quantum materials, and the photonic spin Hall effect (SHE).
Solid-state physics exhibits a family of Hall effects with remarkable physical properties. The classical Hall effect (HE) and the quantum Hall effect (QHE) manifest in systems with time-reversal symmetry broken by the application of a magnetic field. The former results in charge transport transverse to both the magnetic and electric fields applied in the system, while the latter involves topologically protected edge states along with a quantized Hall conductivity. Similar to these effects, Hall physics associated with spin-transport has been also investigated. In the photonic spin Hall effect, particles with distinct spin are driven to opposite directions due to strong spin-orbit interactions in the material. The quantum spin Hall effect (QSHE) is characterized by edge states whose direction of propagation is determined by the spin.
The researchers found that the application of electromagnetic fields allow for active on-demand manipulation of the photonic SHE, which presents a rich dependence with radiation degrees of freedom, material properties, and features nontrivial topological properties. Kort-Kamp demonstrated that the photonic SHE is sensitive to the spin and valley properties of the charge carriers in these materials, showing that spinoptics provides an unprecedented pathway to investigate emergent spintronic and valleytronic phenomena in two-dimensional semiconductors.
Reference: “Topological Phase Transitions in the Photonic Spin Hall Effect,” Physical Review Letters 119, 147401 (2017); doi: 10.1103/PhysRevLett.119.147401. Author: W. J. M. Kort-Kamp.
The Laboratory Directed Research and Development (LDRD) program funded the work, which supports the Lab’s Energy Security mission area and the Materials for the Future science pillar. Technical contact: Wilton Kort-Kamp