STE Highlights, July 2020
Awards and Recognition
Earth and Environmental Sciences
Researchers begin thermal testing for storing high-level nuclear waste in salt formations
Nuclear Engineering and Nonproliferation
New algorithm for special nuclear material detection at border crossings
Awards and Recognition
Ning Xu selected Fellow of the American Chemical Society
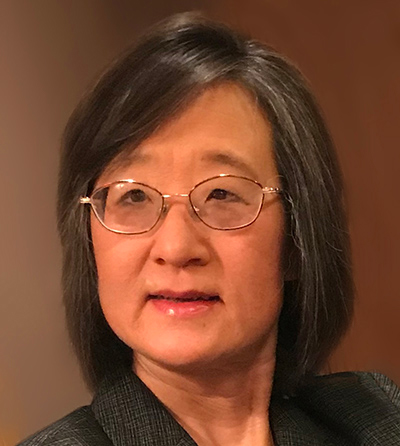
Ning Xu
Ning Xu of the Actinide Analytical Chemistry group (C-AAC) at Los Alamos has been selected as a member of the 2020 class of Fellows of the American Chemical Society (ACS). Xu is being recognized for her sustained contributions to actinide analytical chemistry in support of national nuclear defense, technical nuclear forensics, nuclear material safeguards and deep space exploration.
Xu joined the Laboratory in 2006 after working for the U.S. Environmental Protection Agency National Risk Management Research Laboratory. She is an expert on the analysis of trace elements in plutonium and uranium for nuclear forensics and plutonium pit production. She has received numerous awards for technical innovations that have improved the environmental, safety, and health practices of actinide analytical chemistry.
Xu holds a Ph.D. from the University of Kentucky, a M.S. from the Chinese Academy of Sciences, and a B.S. from the Capital Normal University. Xu has been previously recognized with awards such as the National Nuclear Safety Administration’s Sustainability Award, several Department of Energy Pollution Prevention Awards, and an Outstanding Reviewer Award from the Journal of Radioanalytical Chemistry. She has also served as the chair of the Central New Mexico Local Section of the American Chemical Society. Her Fellows award was announced on July 27th in the ACS journal Chemical and Engineering News .
Technical contact: Ning Xu
Capability Enhancement
New in situ data extraction and visualization capability
Los Alamos researchers developed a new open-source tool as a solution to a longstanding problem: in situ data extraction. Computer scientists as a community have expressed the need for a capability that allows data to be analyzed and visualized while a complicated simulation is running. Previous methods only allowed for post-processing analyses, meaning the scientists had to wait until the simulation finished before analyzing and visualizing the data.
“In situ extraction is a big milestone,” said David Rogers, Visualization and Analytics Project Lead in the Lab’s Applied Computer Science group (CCS-7). “This is important for exascale computing, and it took a combination of research effort and production effort to make it happen.”
The new capability is a combination of Los Alamos-developed tools: ParaView Catalyst, which allows for code integration, and Cinema, which is the visualization framework. Together, these tools offer in situ data extraction and visualization that enable scientists to ask and answer questions previously out of reach.
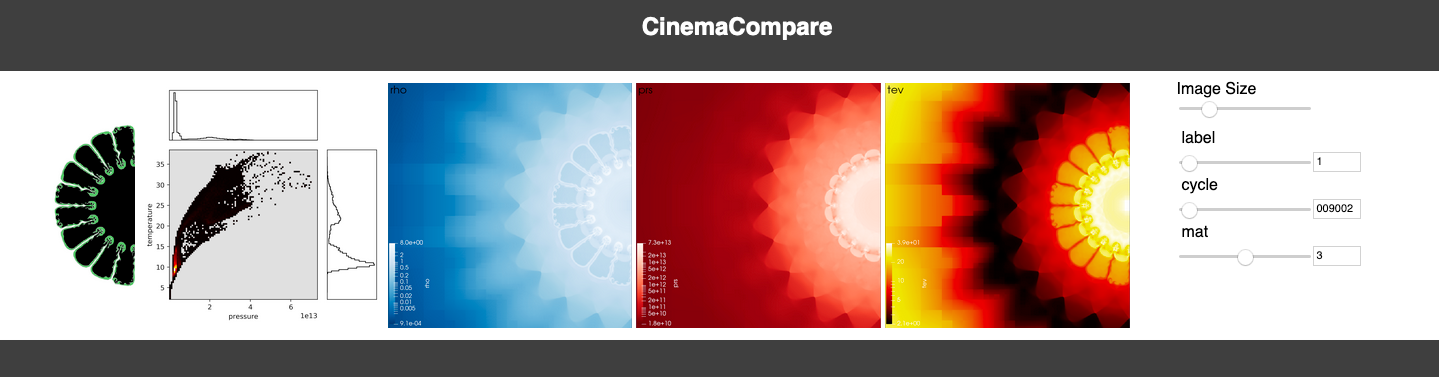
Cinema viewers showing apples-to-apples comparison of different EOS for same simulation. Scientists can use these tools to quickly understand differences between complex simulations. In this example, the tools show clearly that pressure vs temperature is different when only the EOS is changed.
Equation-of-State
One success story of the new capability is comparison of a material by equation-of-state (EOS). The EOS is a mathematical equation that describes a material. Simulation behavior, results, and data can differ for a given material based on varied EOS inputs.
“Los Alamos has a long history of research in EOS as well as a standard way of representing it,” Rogers said. “Our new capability allows scientists to elucidate the impact of a material that varies only in EOS from multiple simulations in situ.”
This is valuable for scientists researching new materials for the U.S. weapons stockpile. Extracted data can highlight the diverging evolution of simulation parameters, including mesh visualizations, material tracking, 2D variable histograms, and 1D/2D plots.
Important for the entire computing community
Beyond its use for Los Alamos weapons designers, the new capability is a huge breakthrough for the greater computing community. That’s why ParaView and Cinema are available to anyone for free on GitHub.
Simulations continue to get bigger and more complex, especially as computer scientists and researchers push to the exascale. These new tools offer flexibility for interpreting that enormous amount of data. It’s fast and simple because the tools are already integrated, offering an infrastructure for anyone that aids in putting visualizations and data extraction into production rapidly.
Now, members from the Visualization and Analytics team can sit with Los Alamos users and ask them what they’d like to visualize. It helps researchers answer questions on the fly.
“We aren’t just handing users a tool,” Rogers said. “We are sitting with them and finding out what kinds of questions they want to ask—questions that haven’t been asked before.”
The new capability has already helped Los Alamos scientists answer real-world problems, and more is certainly coming in the future.
Funding and mission
The Visualization and Analytics Project is currently funded by the Advanced Simulation and Computing program. The DOE Office of Science initiated funding for the project before it was acquired by ASC. The work supports the Laboratory’s Nuclear Deterrence mission area and the Integrating information, Science, and Technology for Prediction and the Weapons Systems capability pillars.
References: T. L. Turton, D. Banesh, T. Overmyer (Purdue University), B. H. Sims, and D. H. Rogers, "Enabling Domain Expertise in Scientific Visualization With CinemaScience," IEEE Computer Graphics and Applications, vol. 40, no. 1, 2020. doi: 10.1109/MCG.2019.2954171.
The open-source Cinema Science project is available on GitHub at github.com/cinemascience. More information can be found on the Cinema Science homepage.
Technical contact: David Rogers
Earth and Environmental Sciences
Researchers begin thermal testing for storing high-level nuclear waste in salt formations
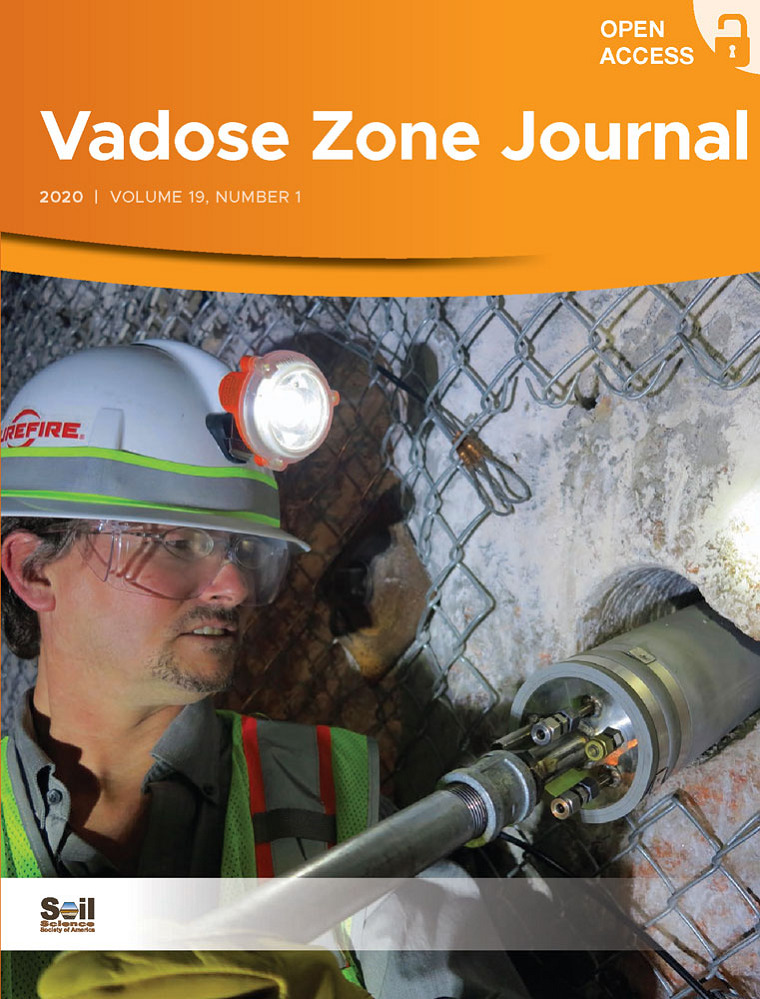
On the cover of Vadose Zone Journal, Philip Stauffer tests the fit of an inflatable packer as part of the Brine Availability Test in Salt (BATS) experiment. This thermal test is being performed 650 m below the surface in the WIPP facility through funding provided by the U.S. Department of Energy.
Can high-level nuclear waste be safely stored in natural salt formations? The answer is unknown, but not for long. Los Alamos researchers in the Earth and Environmental Sciences (EES) Division along with collaborators from Sandia National Laboratories and Lawrence Berkeley National Laboratory designed the Brine Availability Test in Salt (BATS) project to better understand the impacts of high-level waste long-term storage in salt formations.
To simulate high-level waste, a specially designed radiative heater was sent into drilled boreholes at the Waste Isolation Pilot Plant (WIPP)—a natural salt formation currently used for transuranic, low-level, and intermediate-level waste storage.
High-level waste, primarily from spent nuclear fuel (SNF) generated by nuclear power reactors producing copious amounts of energy, remains a challenge to store. In particular, the heat this spent fuel waste generates can complicate long-term (hundreds to thousands of years) salt storage because of the coupled behavior of thermal–hydrological–mechanical–chemical processes in salt.
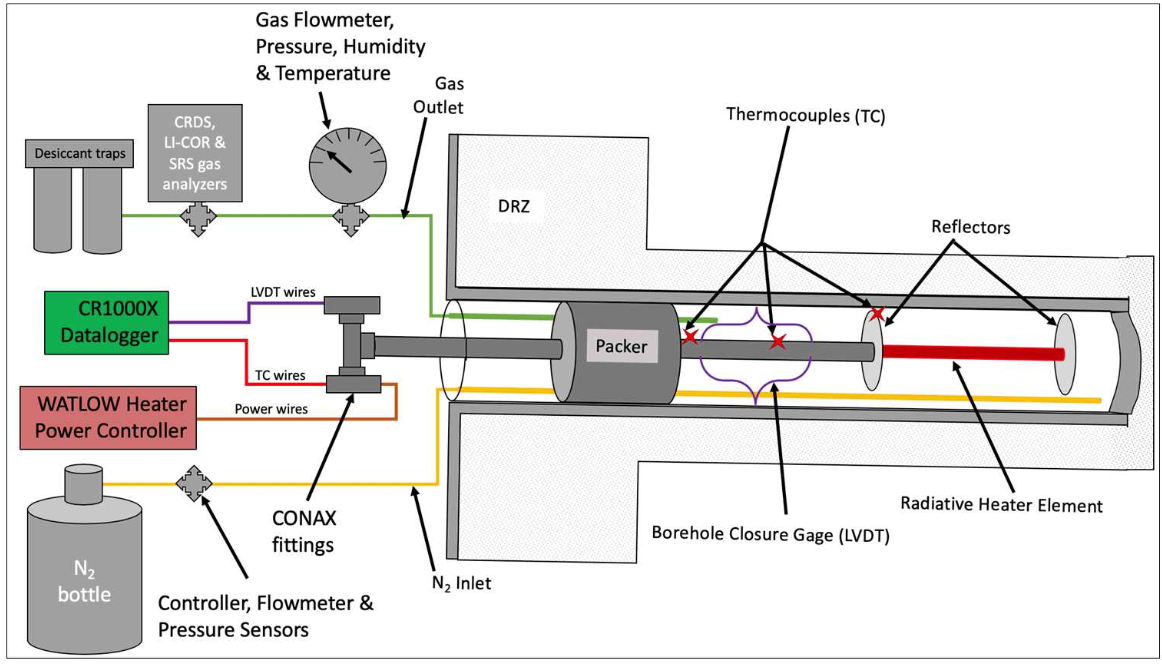
A schematic of a heater design used in the BATS experiments.
BATS Phase 1s
The BATS project is divided into phases. The overall goal of BATS is to bolster the technical basis for disposal of heat-generating waste in salt. The first phase began in June 2018 and ran through May 2019. The results earned BATS phase 1s the cover feature spot in Vadose Zone Journal. Although this was the first experiment in the BATS testing plan, these scientists had already set the research foundations through previous studies. One such study established the multiphase porous flow simulator needed to accurately examine coupled thermal–hydrological–chemical behavior.
“For phase 1s, experimental testing included placing a resistive heater, a 260‐W radiative heater, and a 750‐W radiative heater within previously drilled horizontal boreholes at WIPP while monitoring temperature and water inflow,” said Phil Stauffer of Computational Earth Science (EES-16). “Due to the extremely low permeability of salt, these systems take many years to reach steady state when perturbed by mining activities.”
Time is truly the critical factor in the BATS project. To ensure high-level waste can be safely stored for very long periods of time, the researchers will model and experimentally determine how the heat from this waste will play into the salt environment. For example, the researchers noted damaged rock zones around a borehole significantly affects water inflow and thermal pressurization. Increased pressure around highly radioactive waste could lead to undesirable consequences.
The future of BATS
Follow-on tests are being designed using information gathered from BATS phase 1s. One such follow-on test started running in January 2020. This test uses a heater design that was proven to be robust in phase 1s and is providing detailed data from 28 newly drilled boreholes.
“A primary goal of LANL’s salt repository research is to reduce uncertainty associated with brine availability to repository excavations in salt formations, as part of a larger effort to ensure that we can defend a long-term safety case for disposal of high-level waste in salt,” said Stauffer. “By showing the public that we have a firm understanding of how water moves in salt formations, we will be able to more clearly explain how a salt-based geological waste repository will behave over hundreds to thousands of years.”
Funding and Mission
This work was supported by the Department of Energy Office of Nuclear Energy and the Office of Environmental Management. More information on the project can be found at https://sfwd.lanl.gov/. The research supports the Laboratory’s Energy Security mission area and the Science of Signatures capability pillar.
References:
- Guiltinan, K. Kuhlman, J. Rutqvist, M. Hu, H. Boukhalfa, M. Mills, S. Otto, D. Weaver, B. Dozier, P. Stauffer; “Temperature response and brine availability to heated boreholes in bedded salt,” Vadose Zone Journal. April 2020; https://doi.org/10.1002/vzj2.20019.
- Johnson; S. Otto, D. Weaver, B. Dozier, T. Miller, A. Jordan, N. Hayes-Rich, P. Stauffer; “Heat‐Generating Nuclear Waste in Salt: Field Testing and Simulation,” Vadose Zone Journal. February, 2019; https://doi.org/10.2136/vzj2018.08.0160.
- Johnson, G. A. Zyvoloski, P. Stauffer, “Impact of a porosity dependent capillary function on simulations of porous flow,” Transport in Porous Media. March 2019; https://doi.org/10.1007/s11242-018-1188-x.
BATS Team Members: Hakim Boukhalfa, Eric Guiltinan, Peter Johnson, Doug Weaver, Brian Dozier, Shawn Otto, Nathan Hayes-Rich, Terry Miller, George Zyvoloski, Michelle Bourret, Doug Ware, and Thom Rahn.
Technical contact: Phil Stauffer
Infrastructure Enhancement
Two new research labs open at Los Alamos
The Laboratory opened two new research labs, continuing an effort to improve infrastructure at the main technical area (TA-3). The new Fractured Earth Laboratory is part of the Geochemical Analytical Facility, located next to the Physics Building at TA-3. The Bioscience Research Laboratory is a new 4,000-square-foot building dedicated to biosafety level 2 (BSL-2) research and located between the Materials Science Laboratory (MSL) and Sigma Facility at TA-3, off of Diamond Drive.
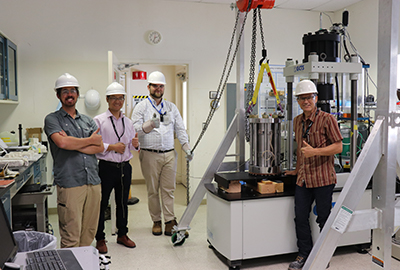
Standing in the new Fractured Earth Laboratory are (l to r) Luke Frash, Wenfeng Li, Nathan Welch, and Bill Carey. The lab is located within the Geochemical Analytical Facility at TA-3.
Fractured Earth Laboratory
The new Fractured Earth Laboratory is a multi-purpose facility offering a high-temperature, high-pressure lab for investigating the mechanisms of flow and transport through fractured materials. The lab (TA03-494-118) is located within the Geochemical Analytical Facility, which is next to the Physics building at TA-3.
Research topics addressed at the lab will cover flow of underground nuclear explosion chemical byproducts through rock fractures, integrity of annular well cement cured at subsurface conditions, multi-scale permeability of fractured shale at subsurface conditions, and fundamental studies of fracture behavior of geomaterials.
Research is already underway at the lab, which is operated by the Earth and Environmental Sciences (EES) division. The new infrastructure strengthens research in support of the Laboratory’s nuclear deterrence and energy security mission areas.
Technical contacts: Bill Carey and Luke Frash
The new Bioscience Research Laboratory at TA-3, located near the Sigma Facility, is dedicated to biosafety level 2 (BSL-2) research.
Bioscience Research Laboratory
The new 4,000-square-foot Bioscience Research Laboratory is dedicated to BSL-2 research. Research topics covered at this laboratory will include host–pathogen studies for understanding disease manifestation, development of human surrogate organ tissue for advanced toxicity and drug experiments, and development of affinity binding molecules for detecting disease or developing therapeutics.
Researchers are expected to move into the lab in August. The Lab is operated by the Bioscience (B) Division. The new infrastructure strengthens research in support of the Laboratory’s Global Security mission area.
Technical contact: Elizabeth Hong-Geller
Materials Science and Technology
Researchers contribute to understanding of microstructure effects on strength of additively manufactured stainless steel
Los Alamos researchers and collaborators added significant experimental results to the understanding of how microstructure affects the properties of additively manufactured 304L-grade stainless steel.
This new data and understanding aids the field of intelligently designed structural materials, which could offer more predictable materials that are less likely to result in catastrophic failure.
The researchers from Los Alamos, the University of New Hampshire, and Argonne National Laboratory compared additively manufactured stainless steel samples to conventionally produced wrought samples. They also investigated the influence of initial microstructure—varied by heat treatment and build orientation—on material properties of strength and martensite formation.
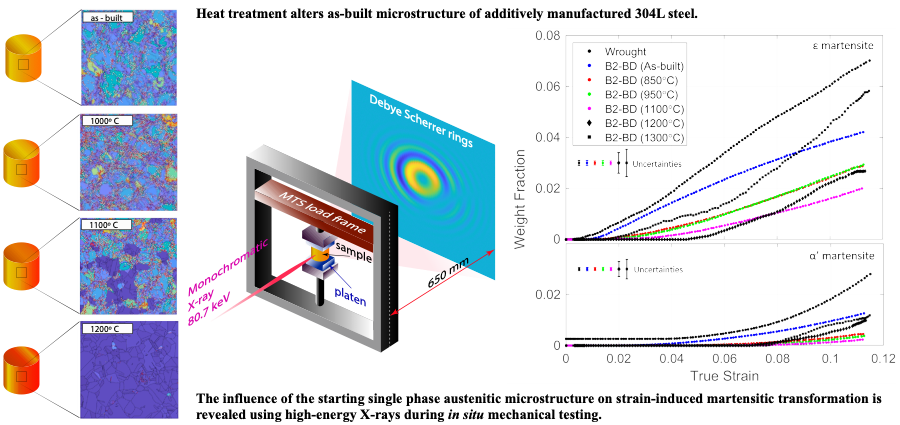
Heat treatment altered the starting additive manufacturing microstructure. This difference resulted in variability in martensitic transformation during compressive deformation. The rate of martensitic transformation decreased for samples treated at temperatures up to 1,100 °C, after which the microstructures recrystallized, resulting in an increased rate of martensitic transformation for those samples treated at higher temperatures.
“Especially during the additive manufacturing process, materials experience extreme thermal conditions locally, which results in unique and non-equilibrium microstructures,” said Reeju Pokharel of Materials Science in Radiation and Dynamics Extremes (MST-8). “The experimental observations linking additive manufacturing microstructure to properties will provide crucial information to modelers.”
Additive manufacturing fills a void
Additive manufacturing provides a promising pathway for the rapid production of near-net-shape engineering parts with complex geometries, which are otherwise impossible or very costly to produce using conventional processing routes. Unlike many conventional methods, additive manufacturing is based on a solid (powder bed, in this case) process rather than a liquefaction process that would require melting of the material. This solid process requires far less energy input than conventional processes.
Although considerable research is being conducted in additive manufacturing, there remains a gap in establishing a process‒structure‒property‒performance relationship in additively manufactured materials. These researchers aimed to flesh out that relationship.
Heat impacts initial microstructure
The researchers designed high-energy X-ray diffraction experiments to be performed at the Advanced Photon Source located at Argonne National Laboratory to study the effect of starting microstructure on martensitic transformation and strength during deformation of 304L-grade stainless steel fabricated via additive manufacturing.
Metallic materials, such as stainless steel, form overwhelmingly uniform crystal microstructures. Defects and dislocations represent a very small portion of the lattice (approximately 0.0001%), but they greatly influence the properties of the material.
The researchers altered the starting microstructure through heat treatment, which created different initial conditions resulting from the selective removal of different aspects of the microstructure. For example, around 1,000°C, the researchers observed restructuring of the initial process-induced dislocations, resulting in the formation of cellular substructures. Upon further heating, these dislocations started annealing out, resulting in the recovery of the material. Past 1,100°C, the initial tortuous grain morphology started evolving and the recrystallization process commenced. Upon recrystallization, equiaxed grain morphology, similar to that observed in conventionally produced wrought or cast materials appeared.
“It was observed that during compressive loading, the formation of strain-induced martensite fractions depended strongly on sample microstructure. The amount of strain-induced martensite was low in samples that showed recovery or retained their original as-built grain structures and high in samples that had recrystallized after heat treatment,” Pokharel said.
While both recovery and recrystallization led to decreased flow strength, change in phase composition had no appreciable effect on the flow stress. The in situ experiments were also performed on wrought samples for comparison. The experimental results provided important understanding of microstructure and property relationships, which are imperative for science-based qualification and certification of additively manufactured materials for engineering applications. Additionally, the experimental observations presented in this work will be crucial for developing and validating physics-based deformation and strength models for additively manufactured materials.
Funding and mission
This work was supported by the Dynamic Materials Properties Campaign (LANL Program Manager Dana Dattlebaum) and is relevant to the DOE’s Dynamic Mesoscale Materials Science Capability (DMMSC). Utilizing the methods developed in this work, future experiments at next-generation light sources with brighter flashes of light and higher data acquisition rates will provide an unprecedented ability to monitor in situ additive manufacturing processes during build and directly link microstructures to properties for a range of elemental metals and alloys, including high-Z materials. The work supports the Laboratory’s Nuclear Deterrence mission area and the Materials for the Future capability pillar.
Reference: “Effects of heat treatment and build orientation on the evolution of ε and α′ martensite and strength during compressive loading of additively manufactured 304L stainless steel,” Acta Materialia (2020), in press. Researchers: Reeju Pokharel, Veronica Livescu, Donald W. Brown, Michael A. Torrez, and George T. Gray III (MST-8, LANL); Nicholas C. Ferreri and Marko Knezevic (University of New Hampshire); Jun-Sang Park (Argonne National Laboratory). https://doi.org/10.1016/j.actamat.2020.04.036
Technical Contact: Reeju Pokharel
Nuclear Engineering and Nonproliferation
New algorithm for special nuclear material detection at border crossings
A new algorithm developed by a Lab researcher and collaborators has the potential to enhance national security at ports of entry. Los Alamos researcher Marc Paff of International Threat Reduction (NEN-3) worked with collaborators from the University of Illinois at Urbana-Champaign, the University of Michigan, Heriot-Watt University (Edinburgh, UK), and the University of Edinburgh to design an algorithm that identifies special nuclear material, including weapons-grade plutonium, in a matter of seconds. Their results were published in Nature Scientific Reports.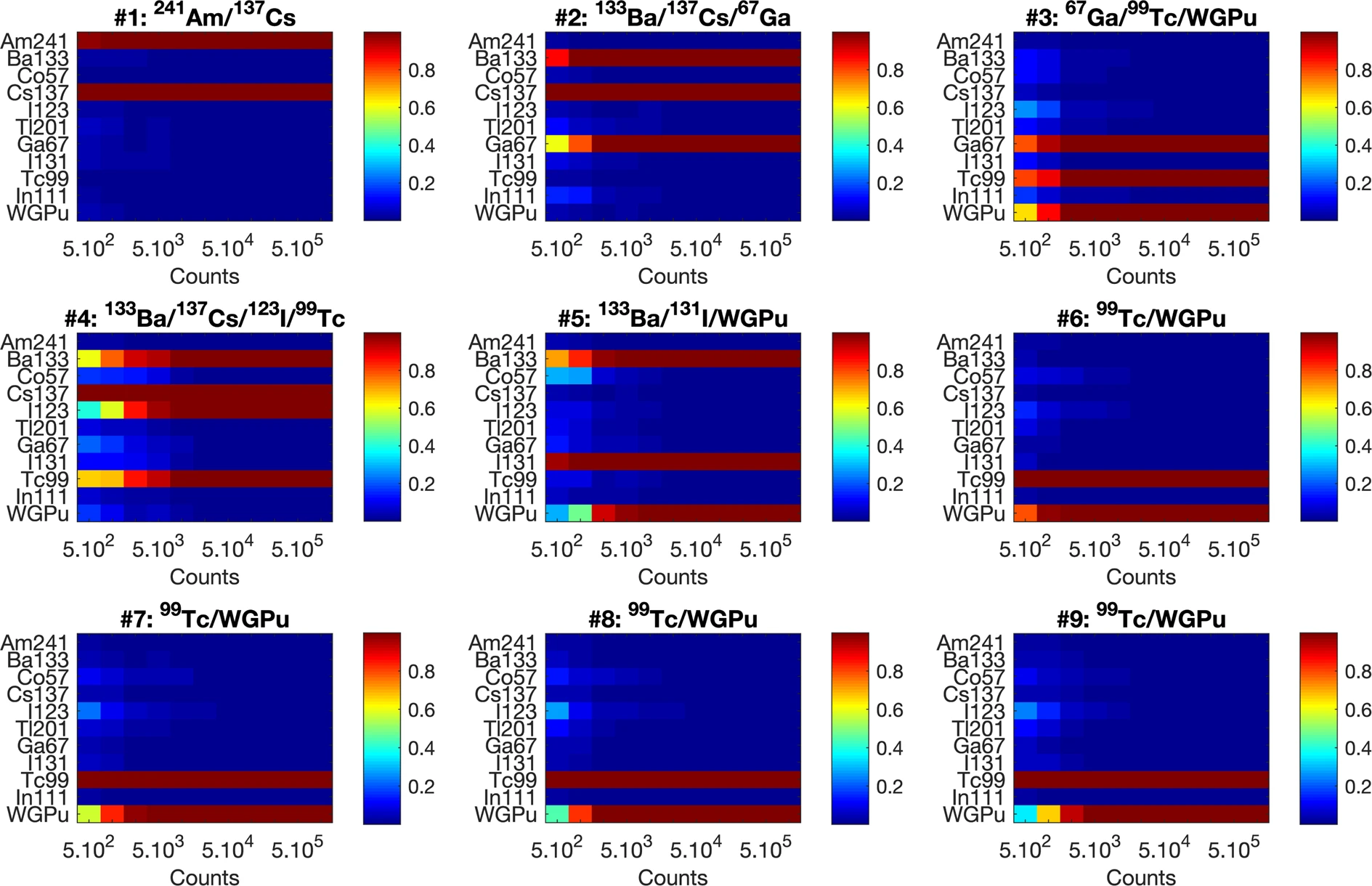
Using the new algorithm, marginal posterior probabilities of the presence of each of the 11 nuclide library sources in a mixture were estimated as a function of the total photon count.
“Our algorithm can potentially improve radiation portal monitor threat discrimination by minimizing ‘nuisance’ alarms produced either by NORM-bearing materials found in shipped cargo—such as ceramics and fertilizers—or radionuclides in recently treated nuclear medicine patients,” Paff said.
Parsing mixed radiation signals has long been a problem for rapid and accurate detection at borders. The stakes are high because smuggling of illicit materials continues to be a threat—one that could have dire consequences for the public if not detected.
Identifying weak radiation signals
Smuggled uranium and plutonium are likely to be encased in radiation-shielding material, rendering the radiation signal weak. This is one example of why a sensitive algorithm is needed in the detection of special nuclear materials. Another common issue is the masking of threat signals by benign radiation signals, such as a small amount of uranium concealed in a large shipment of naturally radioactive fertilizer. Therefore, the question becomes how can weak-signal special nuclear material threats be detected accurately and rapidly at border crossings so as to not inhibit the flow of traffic and not miss any hidden contraband?
The new algorithm, which is based on a Bayesian approach, offers a solution to that decades-long conundrum. Using typical organic scintillation detectors, the team measured 11 nuclides with the new algorithm (MMSEBTG) and two other algorithms. The new algorithm was shown to be more robust than the other two approaches in detecting non-shielded threat materials
“A significant advantage of our algorithm is that it directly provides uncertainty quantification; that is, the estimated probability of the presence of each source from the library of nuclides,” Paff said. These estimates are not directly available for the other two algorithms tested.
The researchers note the new algorithm could be enhanced by coupling it to recently developed spectra reconstruction methods, which would aid in better detection of shielded special nuclear materials.
Funding and mission
This work was funded in part by the Consortium for Verification Technology under Department of Energy National Nuclear Security Administration (NNSA) and the Consortium for Enabling Technologies and Innovation under DOE NNSA. The research supports the Laboratory’s Nuclear Deterrence mission area and the Complex Natural and Engineered Systems and the Science of Signatures capability pillars.
Reference: Altmann, Y., Di Fulvio, A., Paff, M.G. et al. Expectation-propagation for weak radionuclide identification at radiation portal monitors. Sci Rep 10, 6811 (2020). https://doi.org/10.1038/s41598-020-62947-3
Related article: Catching nuclear smugglers: fast algorithm could enable cost-effective detectors at borders https://npre.illinois.edu/news/36509
Technical contact: Marc Paff
X-Computational Physics
LANL releases open-source data remapping software Portage 2.2
A Los Alamos-developed software package targets a longstanding mathematical problem in computing—data remapping. This task involves moving data from one computational mesh (which represents a geometric object) to another mesh. The remapping becomes particularly complicated if those meshes are not geometrically aligned. Another common data movement task involves moving data between physics codes, which often cover disparate physical regimes.
Portage 2.2, an open-source data remapping software, is a streamlined solution to these persistently challenging problems.
“We saw that each code team was having to expend significant effort on remapping or linking, so we decided to make a standalone package dedicated just to this,” said Angela Herring, Portage lead, in the Lab’s Lagrangian Codes group (XCP-1).
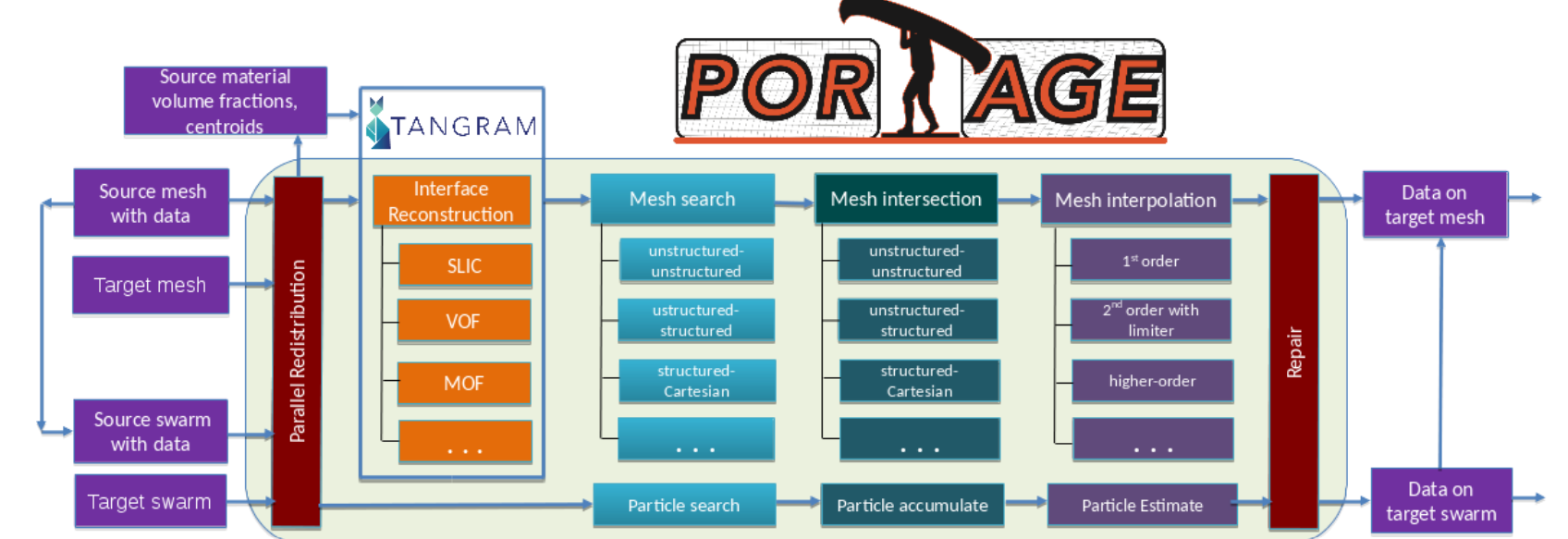
An overview of how Portage works as a framework for the transfer of data between meshes and particle swarms in computational physics applications.
Why is data movement so complicated?
The data on a computational mesh encompasses many physical quantities, such as momentum and mass. These quantities must be conserved when moving data to a new location, or mesh. Although conservation is a challenge—one that requires specialized developers—mapping onto a mesh that isn’t exactly the same as the original mesh greatly compounds the issue. Often, the boundaries of the source and target meshes in simulations don’t match up precisely. This may happen because curved boundaries are discretized with different resolutions in the two meshes or because two physics packages view the geometry of the domain a bit differently.
“Remapping happens over and over.” Herring said. “This can be very computationally expensive.”
Portage 2.2, which was released in June as open-source software, offers efficient, scalable remapping. This becomes particularly important for exascale computing.
Why is data movement so important?
The U.S. Department of Energy has tasked several national laboratories with creating the foundations for exascale computing. At Los Alamos that project is called Ristra and it deals with massive amounts of data from extreme-scale multi-physics simulations. The idea for Portage came from Ristra’s need for accurate and swift remapping.
“A key feature that we wanted for Portage was reusability. That’s really the Holy Grail of open-source sharing,” Herring said.
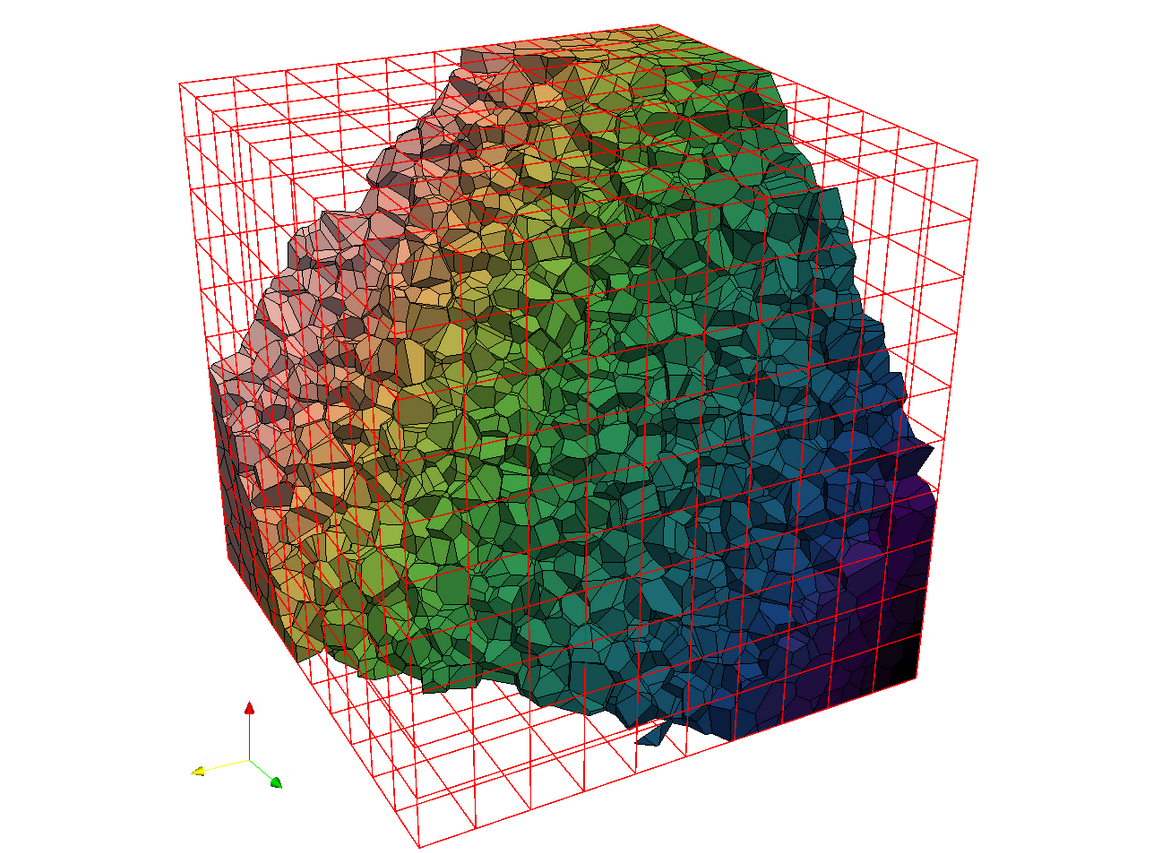
An example of visualizing a source mesh (red) and part of a target mesh (black) with remapped data using the Portage app Jali, app/portageapp/portageapp_jali.cc.
Not only is Portage reusable, offering remapping and code linking in large simulation codes such as xRAGE, Portage also offers scalability and compatibility with existing and new computing architectures. This facilitates the rapid adoption of this new software.
Portage 2.2 offers additional features beyond the original remapping concept. These new features include a novel part-by-part remap, swept-face remap, a prototype momentum-conserving remap, and various performance improvements. The Portage team continues to innovate and add to this successful new package.
Funding and mission
The Ristra project is funded by the National Nuclear Security Administration’s Advanced Simulation and Computing Program. The work supports the Laboratory’s Nuclear Deterrence mission area and the Weapons Systems and Integrating Information, Science, and Technology for Prediction capability pillars.
Software release pages: Ristra Portage https://github.com/laristra/portage and https://laristra.github.io/portage/
Portage team members: Angela Herring, Christopher Malone, Daniel Shevitz, Evgeny Kikinzon, Hoby Rakotoarivelo, Jan Velechovsky, Konstantin Lipnikov, Navamita Ray, Rao Garimella, and Mikhail Shashkov.
Ristra project leads: Aimee Hungerford and David Daniel
Technical contacts: Angela Herring and Aimee Hungerford