STE Highlights, August 2020
Awards and Recognition
Bioscience
Fine-tuning light harvesting antenna for improved photosynthesis boosts algae biomass three-fold
Earth and Environmental Sciences
Historical data leads to better modeling for radioactive gas seepage from underground nuclear tests
High Performance Computing
LANL partners with Eideticom to bring computational storage processor to the market
Materials Physics and Applications
Experimental demonstration of advanced photonic systems leads to boosted performance
X-Computational Physics
New record for highest pressures ever achieved in laboratory equation-of-state measurements
Awards and Recognition
Burns receives ACS Francis P. Garvan‒John M. Olin Medal
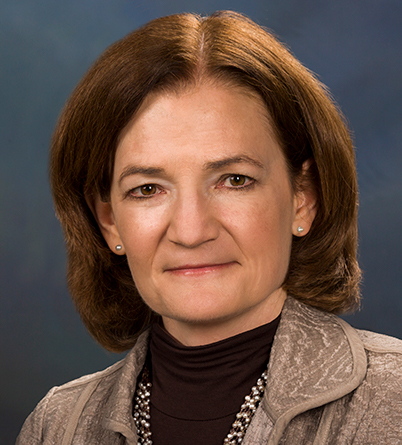
Carol Burns
Carol Burns, Executive Officer for the Deputy Director for Science, Technology & Engineering (DDSTE), was selected as the recipient of the 2021 American Chemical Society’s (ACS) Francis P. Garvan‒John M. Olin Medal. This national award recognizes distinguished service in the field of chemistry by female chemists.
Burns will be honored with the medallion at the ACS 2021 Spring National Meeting on March 23, 2021, in San Antonio, Texas. The award was established in 1936, recognizing one top female chemist each year.
“Carol has been a leader not only in her field of chemistry as well as all of science, technology and engineering but also as a mentor, role model and inspiration to the next generation of women scientists for many years,” said John Sarrao, Deputy Director for STE. “This is a very well-deserved award.”
Burns is an expert in actinide chemistry. She was named a Laboratory Fellow in 2003 and has supported the role of chemistry in national security throughout her career. While contributing to nuclear security programs, she has maintained a strong and vibrant research program in actinide coordination and organometallic chemistry. She pioneered the development of an entirely new class of high-valent uranium compounds containing metal-ligand multiple bonds, supported by cyclopentadienyl ligands. This work has contributed substantially to the understanding of the electronic structure of the early actinides, and inspires current work in the field.
In her management positions at Los Alamos, Burns has championed workforce development and gender diversity issues. She has formally and informally mentored numerous students, postdocs, and early career scientists at LANL. She was recognized by LANL’s Women’s Diversity Working Group with their Women’s Career Development Mentoring Award. Externally, she has served on advisory committees for universities and other national laboratories, and served in fellowship organizations at the graduate and postdoctoral level, including the Hertz Foundation and the Washington Research Foundation.
In her current role in DDSTE, she supports the Deputy Director and Director’s Office in oversight of line and program organizations in Chemistry, Earth, and Life Sciences; Global Security; Physical Sciences; and Simulation and Computation. She coordinates the development and integration of strategy for science, technology and engineering at the Laboratory and has oversight of the associated institutional investments.
Burns received her B. A. in Chemistry from Rice University, and her Ph.D. in Chemistry as a Hertz Foundation Fellow at the University of California at Berkeley. She came to LANL as a J. Robert Oppenheimer Postdoctoral Fellow.
Lebensohn and team win Additive Manufacturing Modeling Challenge
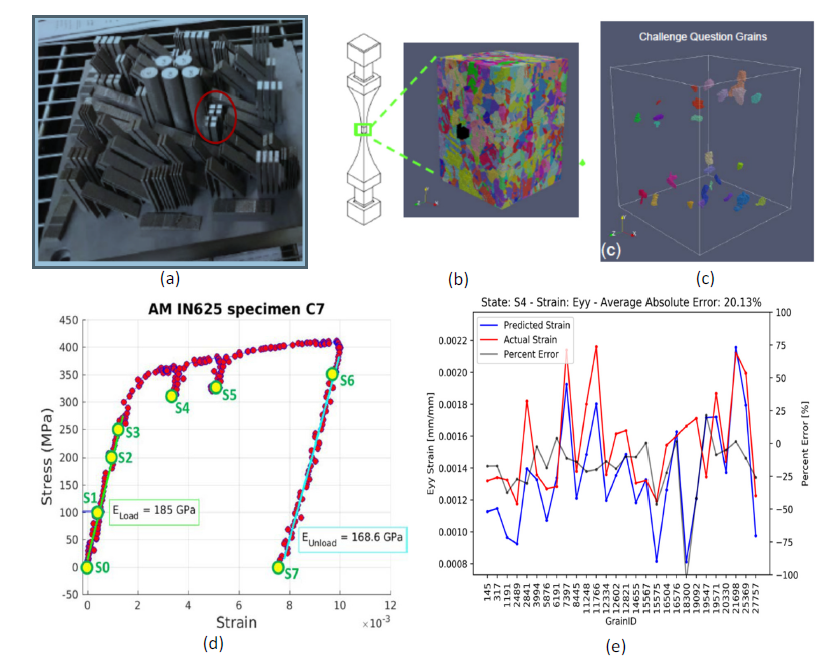
(a) The 3D-printed IN625 parts. (b) HEDM sample and initial grain structure. (c) Locations of the 28 grains involved in the challenge. (d) Macroscopic stress-strain response and states at which grain-averaged elastic strains were measured. (e) Comparison between predicted and (a posteriori disclosed) measured elastic strains (longitudinal component) of the 28 challenge grains, for state S4.
Ricardo Lebensohn of Fluid Dynamics & Solid Mechanics (T-3) along with team members from the University of Utah and Carnegie Mellon University were awarded first place in the Air Force Research Laboratory and America Makes Additive Manufacturing Modeling Challenge, under the category “Micro-scale Structure-to-Property Predictions.”
The challenge called for innovative modeling approaches to advancing additive manufacturing (AM) technologies. Challenge participants were provided datasets needed to obtain predictions that test the accuracy of micromechanical models.
Lebensohn and the team were cited for best blind prediction of grain-average elastic strains within a 3D-printed and subsequently deformed specimen of the nickel–chromium alloy INCONEL 625 (IN625), which was measured in situ by High-Energy Diffraction Microscopy (HEDM) in the Advanced Photon Source (APS).
“Winning this challenge shows the ability and agility of LANL’s modeling tools to impact the emerging technology of additive manufacturing of metallic parts,” Lebensohn said.
Given an explicit representation of the initial microstructure and the macroscopic stress-strain behavior of an AM IN625 sample, the winning team predicted local elastic strains for specified grains at specified macroscopic loading points using LANL’s elasto-viscoplastic fast Fourier transform (EVPFFT) code. The average error of the blind predictions versus measurements was nine percent.
The EVPFFT code, which was vital to making accurate predictions, was created by Lebensohn through the support of LANL’s Laboratory Directed Research and Development (LDRD) Program and the DOE-DoD Joint Munitions Program.
Related article: America Makes and Air Force Research Laboratory Announce Awardees of the Additive Manufacturing Modeling Challenge Series
Technical contact: Ricardo Lebensohn
MOSAiC team honored with DOE Service Award
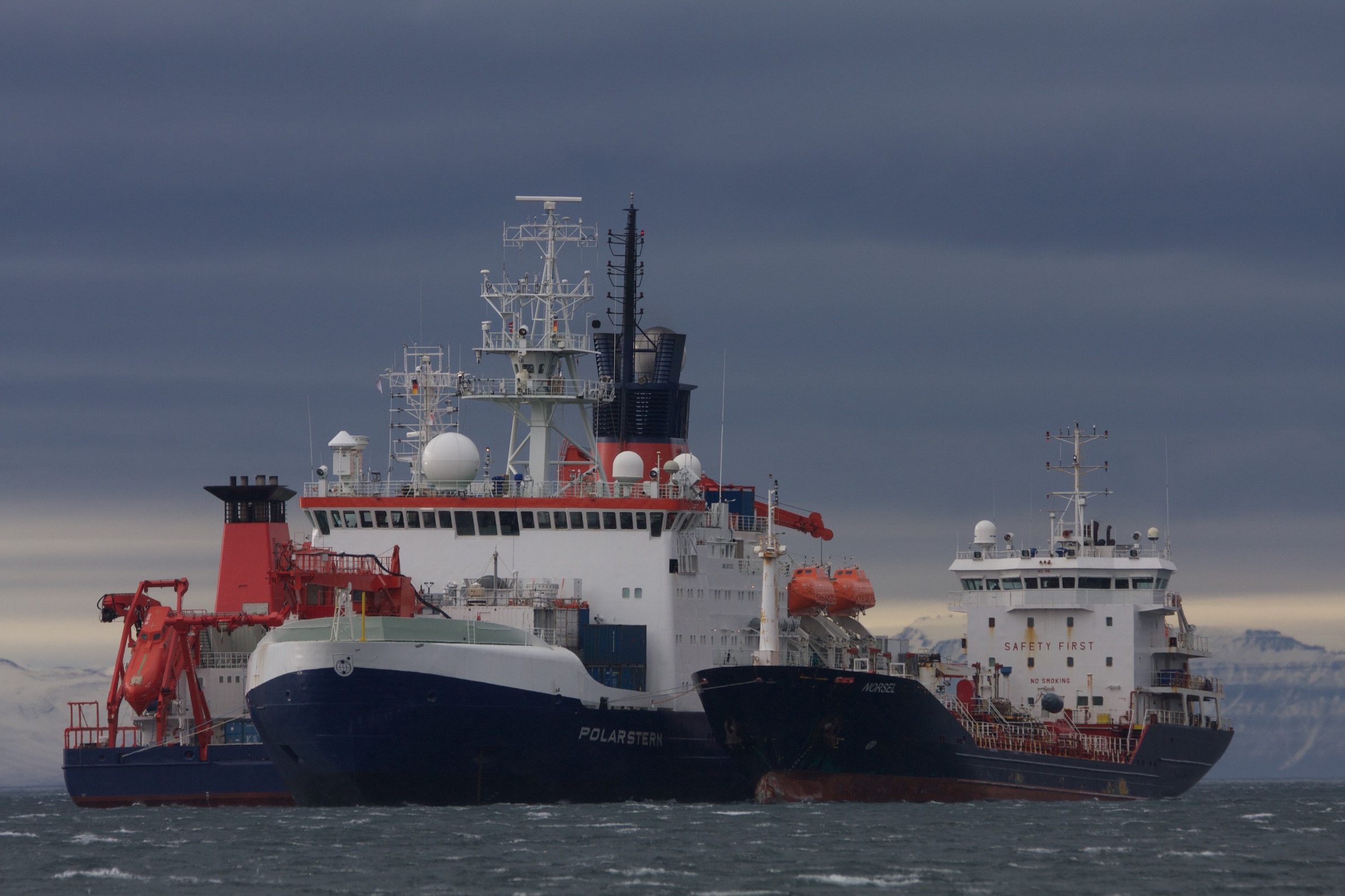
The R/V Polarstern (left) helps carry out the crew exchange and supply delivery for MOSAiC Leg 4 in early June 2020 near Svalbard, Norway. Photo credit: Leonard Magerl, Alfred Wegener Institute.
The Multidisciplinary Drifting Observatory for the Study of Arctic Climate (MOSAiC) deployment team received a 2020 Department of Energy (DOE) Service Award for their exemplary work and service to the Atmospheric Radiation Measurement (ARM) national user facility community.
Led by David Chu, Earth System Observations (EES-14), the team has been aboard the Polarstern icebreaker for nearly a year. The expedition will conclude in late October at a port in Germany. The full deployed team includes John Bilberry, Matt Boyer, Zoé Brasseur, Vagner Castro, Jody Ellis, Dean Greenamyer, Steele Griffiths, Todd Houchens, Wessley King, Misha Krassovski, Paul Ortega, Tercio Silva, Janek Uin, and Juarez Viegas.
Additionally, Brad Perkins (EES-14) was honored with a DOE Service Award for his system development and maintenance expertise, ingenuity, collaborative nature, and dedication to ARM’s mission. Perkins is part of the operations support tools team, which is based in Los Alamos.
For more about the MOSAiC expedition, see the DOE ARM updates.
El-Atwani and Uberuaga recognized as Outstanding Reviewers for the Acta Journals
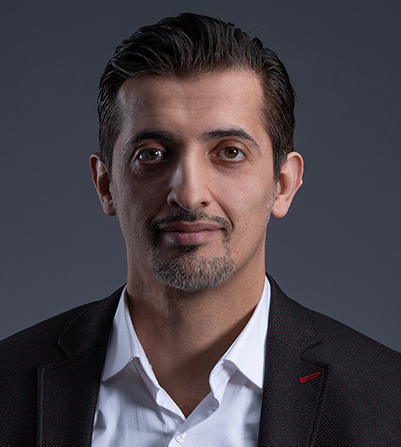
Osman El-Atwani
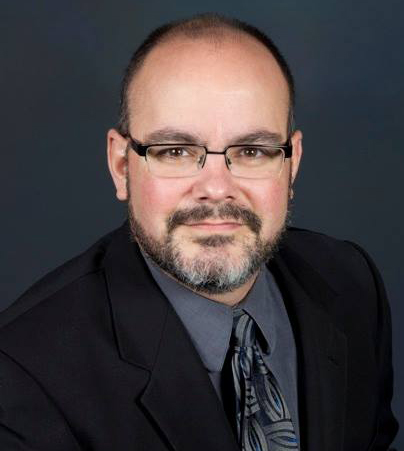
Blas Uberuaga
Osman El-Atwani and Blas Uberuaga, both of Materials Science in Radiation & Dynamics Extremes (MST-8), were recognized by the Acta Journals Acta Materialia and Scripta Materialia as 2020 Outstanding Reviewers for their work in 2019.
The Acta Journals are published through Elsevier, and both deem peer review as the cornerstone of science. These reviewers help maintain continued high quality publications for the journals. Together, Acta Materialia and Scripta Materialia have an impact factor over 7.6; it has consistently climbed over the past few years.
El-Atwani and Uberuaga understand the importance of ensuring the integrity and quality of materials science publications.
“I admit that reviewing papers is not one of my favorite parts of this job, but it is one of the most critical,” Uberuaga said. “Peer review is central to building trust in the science we generate. I'm honored to be recognized for my contributions.”
Similarly, El-Atwani is grateful to be recognized for the second time in the last three years. “Peer reviewing others’ works fairly and promptly is an important quality of a researcher. As an imperative part of my research enthusiasm and responsibilities, I am glad to contribute to this goal,” El-Atwani said.
Technical contacts: Osman El-Atwani and Blas UberuagaBioscience
Fine-tuning light harvesting antenna for improved photosynthesis boosts algae biomass three-fold
Los Alamos bioscience researchers along with collaborators discovered a way to improve photosynthesis efficiency in algae. The hope was that this would lead to greater biomass yields, and it did, sometimes up to a three-fold increase in biomass yield.
Biomass yield is one of the main limiting factors in achieving widespread use of algae as a source for clean, sustainable, and renewable fuel. A key benefit of algae is production without the need for arable land, which is increasingly limited.
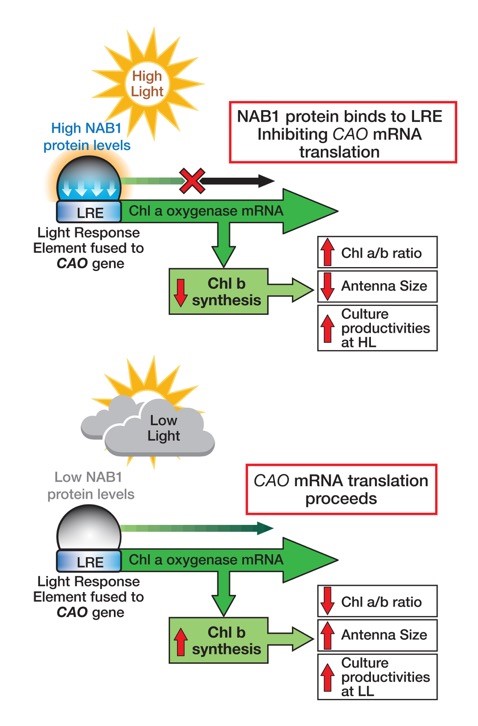
Adjusting the antenna size depending on light availability boosts biomass yield in algae.
“There are several metabolic targets that can be modified to improve photosynthetic efficiency,” said Los Alamos bioscientist Sangeeta Negi of Bioenergy and Biome Sciences (B-11). Those targets include altering the light-harvesting antenna size of the algae through genetic manipulation of the CAO gene.
Antenna modulation is needed to improve efficiency
The researchers noted natural algae photosynthesis efficiency is quite low, only 1–5% efficient. However, theory indicates efficiency of up to 11% should be possible. It seems nature is keeping efficiency balanced with the risk for damage, as too much pent-up photo-energy can backfire. The researchers turned to genetic mutation to boost efficiency without boosting detrimental effects.
The goal was to design algae that could dynamically adjust their peripheral light-harvesting antenna size in response to changing light intensities. That means the antenna size would increase with low light levels and decrease with high light levels. To achieve this adjustability feature, the researchers modulated the expression of the CAO gene by adding a light response element to it. This light-sensitive element essentially switches the gene on and off, depending on light intensity.
Biomass yield improvement is significant
The algae that could adjust their light-harvesting antenna showed 1.8 to three-fold greater biomass yields than the wildtype algae, even in low light. This value is significant for a few reasons. For one, it is among the largest improvements in biomass yield to date. Second, it indicates that in natural algae growing conditions, which experience changing light with time and depth of culture density, the adjustable algae can still produce greater yields. Therefore, this approach is practical and realistic.
These biomass accumulation rates meet the requirements for needed algal biofuel price points to be competitive with petroleum.
Funding and Mission
This work was supported by DOE programs: Photosynthetic Antenna Research Center (BES-PARC), Realization of Algae Potential (BETO-REAP), Producing Algae for Coproducts and Energy BETO-PACE, National Alliance for Advanced Biofuels and Bioproducts (BETO-NAABB). It was also supported by Enterprise Rent-a-Car Institute for Renewable Fuels; Donald Danforth Plant Science Center; U.S. Air Force‐Office of Scientific Research; Japan Society for the Promotion of Science and the Ministry of Education, Culture, Sports, Science and Technology, through the Network of Centers of Carbon Dioxide Resource Studies in Plants; LANL Laboratory Directed Research and Development (LDRD); the New Mexico Consortium; Pebble Laboratories; and the University of Illinois at Urbana‐Champaign.
The research supports the Laboratory’s Energy Security mission area and the Complex Natural and Engineered Systems capability pillar.
Reference: Sangeeta Negi (LANL), Zoee Perrine (Donald Danforth Plant Science Center), Natalia Friedland (Stanford University), Anil Kumar (Donald Danforth Plant Science Center), Ryutaro Tokutsu (Japan Science and Technology Agency), Jun Minagawa (Japan Science and Technology Agency), Howard Berg (Donald Danforth Plant Science Center), Amanda N Barry (LANL, now SNL), Govindjee Govindjee (University of Illinois at Urbana-Champaign), Richard Sayre (New Mexico Consortium). “Light Regulation of Light-Harvesting Antenna Size Substantially Enhances Photosynthetic Efficiency and Biomass Yield in Green Algae.” The Plant Journal. 2020 Mar 17. doi: 10.1111/tpj.14751
Collaborating institutions: New Mexico Consortium, Donald Danforth Plant Science Center, Division of Environmental Photobiology, National Institute for Basic Biology, Okazaki, Japan, Department of Basic Biology, School of Life Science, The Graduate University for Advanced Studies (SOKENDAI), Okazaki, Japan, CREST (Core Research for Evolutional Science and Technology), Japan Science and Technology Agency (JST), Okazaki, Japan, Department of Biochemistry, Department of Plant Biology, Center of Biophysics and Quantitative Biology, University of Illinois at Urbana-Champaign.
Technical contact: Sangeeta Negi
Computer, Computational and Statistical Sciences
New parallel programming system, Legion, for the exascale
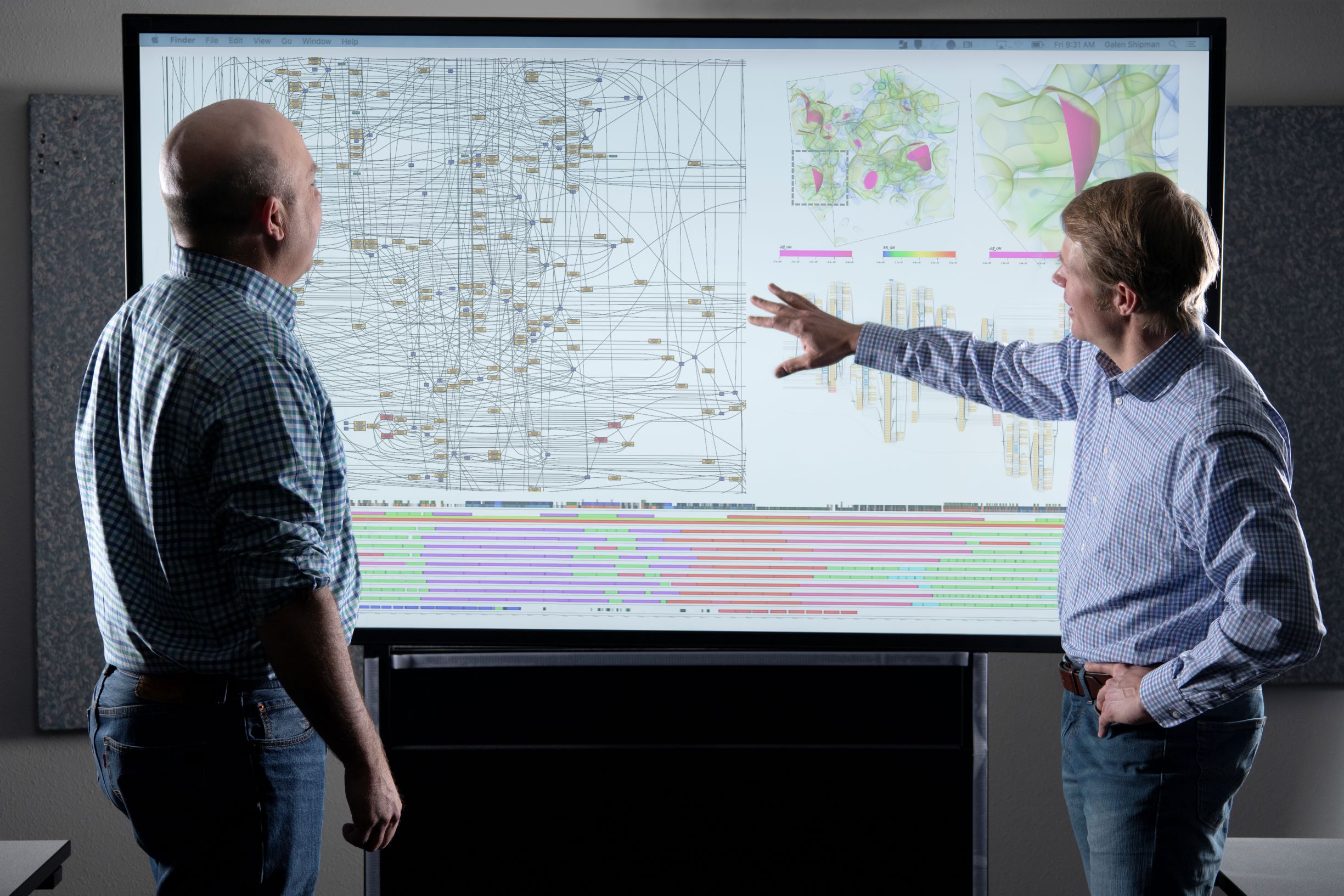
Legion, an open-source software, automates complex scheduling of tasks and data movement in supercomputing applications.
Los Alamos researchers in Computer, Computational, and Statistical Sciences along with several collaborating institutions released the newest version of their parallel programming system, called Legion.
“Supercomputers are becoming more complex, and as we move toward computing at the exascale, we need to be able to automate more of the complexity of programming for these systems,” said Galen Shipman, Applied Computer Science (CCS-7).
That is precisely what Legion does: automates complex scheduling of tasks and data movement in supercomputing applications. Before Legion, these time-consuming responsibilities fell to application developers. As supercomputing applications become more complex and data exponentially increases, placing the burden of these tasks on application developers becomes unsustainable.
“Building upon the state-of-the-art in programming models research, Legion was designed from the ground up to enable complex applications to take full advantage of modern exascale systems,” said Pat McCormick, Applied Computer Science (CCS-7).
Legion is open-source and will be used in LANL’s next-generation supercomputer Crossroads.
Applications can be written in Regent
Legion provides a programming language called Regent. It was created specifically for the Legion system as a high-productivity language that is simple to read and write. Regent enables application developers to write a computational task once (single source), and the Regent compiler then generates optimized variants of tasks for a variety of supercomputing architectures. This enables an application to run on multiple computing devices, such as CPUs or GPUs, without changing the source code.
Regent allows the application developer to write each task in sequence but uses Legion to run those tasks in the most efficient way possible, which is likely out of order. Regent builds on top of Legion’s task analysis capability to discover task parallelism (determining which tasks can run simultaneously) in sequential code by computing a dependence graph over tasks.
Legion improves applications on supercomputers
The Legion team has been perfecting the system for years, and with each new version, more people are adopting the technology. The newest release was Version 20.06. It comes with a long list of users, which include applications such as the Soleil-X multi-physics application from Stanford, the S3D compressible reacting flow Direct Numerical Simulation code from Sandia National Labs, a near real-time data analysis framework for the Linac Coherent Light Source at SLAC National Accelerator, a deep-learning framework used for cancer research in a partnership of National Institutes of Health and the Department of Energy, training of deep neural networks at Facebook, and many more.
With Legion, these applications run faster and more efficiently. Performance improvements of 10X have been achieved across a number of these applications. More applications are turning to Legion as they look to achieve performance on modern supercomputers.
“Although traditional methods are likely to remain for some, those who wish to compute at the exascale and beyond will undoubtedly see the benefits of Legion,” Shipman said.
Funding and mission
Legion is funded by the DOE National Nuclear Security Administration – Advanced Simulation and Computing Program and the DOE Office of Science – Advanced Scientific Computing Research. The work supports the Laboratory’s Nuclear Deterrence mission area and the Integrating Information, Science, and Technology for Prediction capability pillar.
Collaborating institutions: Stanford University, NVIDIA, SLAC National Accelerator, UC Davis, Sandia National Laboratories.
Relevant Legion links: Legion information ; Download Legion from GitHub
Technical contact: Galen Shipman
Earth and Environmental Sciences
Historical data leads to better modeling for radioactive gas seepage from underground nuclear tests
Researchers from Earth and Environmental Sciences (EES-16) discovered parameters that better predict whether an underground nuclear explosion will lead to late-time radioactive gas seepage. Not all explosions result in radioactive gases pushing to the ground surface.
Using historical U.S. nuclear test program data from 16 underground nuclear explosions with similar geology and test setup, the researchers analyzed discriminating factors that heavily impact whether an underground nuclear explosion will result in radioactive gas seepage.
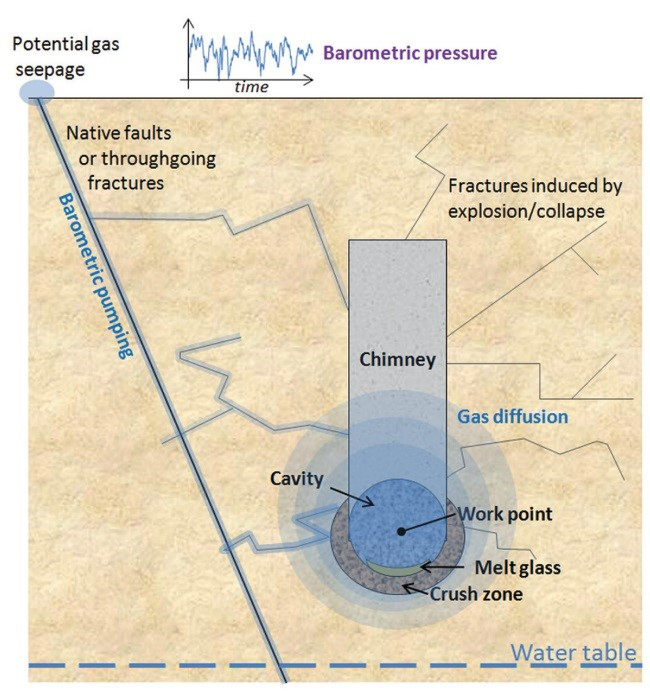
Conceptualization of subsurface damage and gas migration pathways to the ground surface by barometric pumping after an underground nuclear explosion (from Jordan et al., 2014).
Verification of test ban treaties
Detecting, confirming, and understanding underground nuclear explosions is of vital importance to national security. This new research will aid in the verification of nuclear test ban treaties.
Detecting radioactive gases seeping into the atmosphere can provide “smoking gun” evidence of an underground nuclear explosion. These explosions generate a suite of radioactive gases, including noble gases that do not interact easily with other materials and some of which decay slowly enough to persist until transported to the ground surface over days to months, a process known as late-time seepage. Detection of particular noble gases with specific isotopic ratios seeping into the atmosphere from a suspected test site can positively identify the occurrence of a nuclear explosion when little other evidence is available.
Factors that predict late-time seepage
The rate at which radioactive gases seep from underground nuclear explosions to the ground surface depends on the geology, atmospheric conditions, and the nuclear device and its yield. The researchers used subsurface gas transport models that account for these factors to understand how radioactive gases move from the depth of the nuclear explosion to the ground surface.
The researchers selected 16 historical underground nuclear explosions that occurred at the Nevada National Security Site (NNSS): five with late-time releases and 11 without. Potential factors that could impact late-time gas seepage include: (1) post-explosion surface damage, (2) proximity to faults, (3) barometric pressure trends before and after the test date, (4) gas seepage soil properties (permeability and air-filled porosity), and (5) geologic stratigraphy. The importance of these factors was evaluated by the researchers using the historical data along with numerical and analytical modeling.
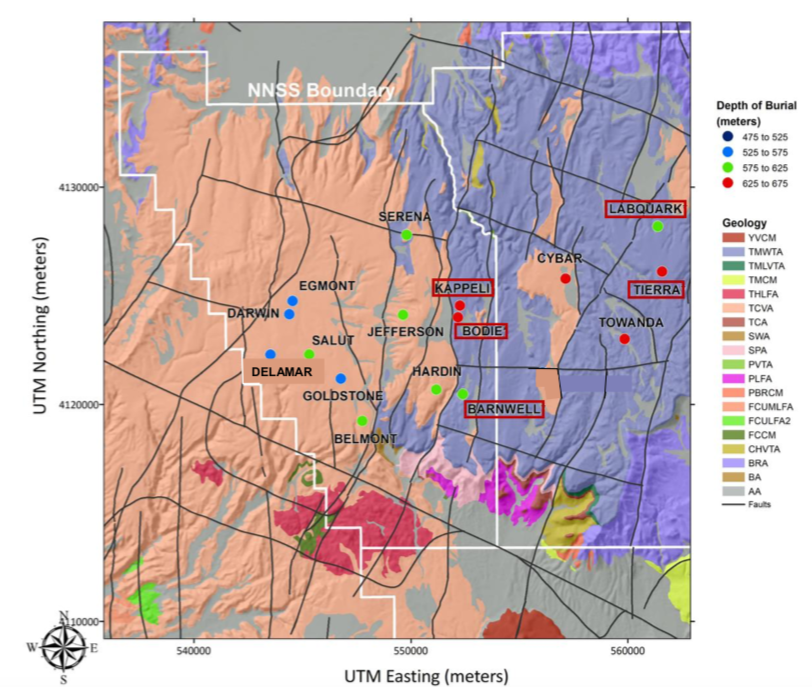
Map of the surficial hydrostratigraphy of Pahute Mesa (NSTec, 2014) with underground nuclear explosion locations. Black lines indicate faults and white lines indicate NNSS boundaries and operational areas (Area 20 on left Area 19 on right). Red boxes indicate where late-time seeps were observed.
A key finding of the research is the amount of air space available in the rock (air-filled porosity) is inversely correlated with whether an underground nuclear explosion would result in late-time seepage. Less air-filled porosity increases the potential for late-time seepage. Another key finding is the time of year when the underground nuclear explosion occurs is important. This is because barometric pressure trends change seasonally, with larger variations during the fall and winter. Therefore, explosions conducted in the fall and winter at the NNSS (when barometric gas transport efficiency is higher) are more likely to have late-time seeps.
Surprisingly, neither the severity of post-explosion surface damage nor proximity to existing natural faults with surface expressions are strong indicators of late-time seeps. Numerical and analytical models are able to discriminate between underground nuclear explosions resulting in late-time seeps and those without. However, the numerical models did not accurately predict the exact timing of the releases. Analytical modeling indicated that knowledge of rock fracture widths created by the explosions (information not available at all 16 explosions analyzed) would improve the identification of underground nuclear explosions resulting in late-time seeps.
Funding and mission
The research was supported by the National Nuclear Security Administration Office of Defense Nuclear Nonproliferation Research and Development. The work supports the Laboratory’s Nuclear Deterrence mission area and the Science of Signatures capability pillar.
References:
Bourret, S. M., Kwicklis, E. M., Harp, D. R., Ortiz, J. P., Stauffer, P. H. 2020. “Beyond Barnwell: Applying lessons learned from the Barnwell site to other historic underground nuclear tests at Pahute Mesa to understand radioactive gas-seepage observations.” J. Environ. Radioact. 222C. https://doi.org/10.1016/j.jenvrad.2020.106297
Harp, D. R., Bourret, S. M., Stauffer, P. H., and Kwicklis, E. M. (2020).“Discriminating underground nuclear explosions leading to late‐time radionuclide gas seeps.” Geophysical Research Letters, 47, e2019GL086654. https://doi.org/10.1029/2019GL086654
Technical Contacts: Michelle Bourret and Dylan HarpEducational Outreach
Quantum Computing Summer School goes virtual for the first time
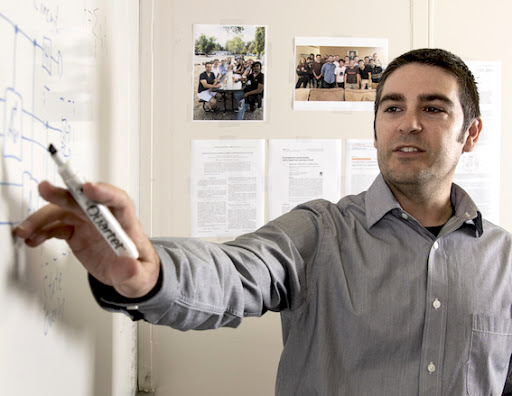
Patrick Coles initiated the Quantum Summer School three years ago, with interest and applicants growing each year.
The Los Alamos Information Science and Technology Institute (ISTI) hosted its 10-week Quantum Computing Summer School fully online for the first time this summer. With the idea that leadership begets leadership, the summer school is led by three top Los Alamos quantum scientists — Patrick Coles and Lukasz Cincio of Physics of Condensed Matter and Complex Systems (T-4), and Carleton Coffrin of Information Systems and Modeling (A-1) — and includes lectures from guest experts in academia and industry.
“One of our goals is to establish a pipeline,” Cincio said, “drawing the top students from around the world to Los Alamos.”
That goal had to be adapted in 2020 because of the COVID-19 pandemic. In all, 18 students ranging from upper-level undergraduates to Ph.D. candidates participated in the summer school virtually instead of coming to Los Alamos in person.
“I felt saddened not getting to work at the historic Lab itself and meeting all the talented people in the program in person,” said Jacob Beckey, a student from the University of Colorado. “However, my mentors were all quick to respond, and we had virtual coffee breaks that substituted some of that in-person interaction we would have gotten.”
Overall, the program was able to run in full, not needing to cut any lectures or projects as a result of virtual tutelage. This was quite an accomplishment given that 2020 represented the largest group of students the co-leads have ever taught.
“If we have to run this again virtually, we know we can,” Coles said. “But we are all looking forward to an in-person experience next year.”
There will be some positive and lasting changes from having executed this summer camp as a fully remote experience, including recording lectures for the first time. These will be posted to the ISTI site and open to the public, fulfilling a common request from those unable to participate in the rather selective summer school. This year’s group of 18 students was chosen from a pool of 400 applicants.
The co-leads made their student selections with priority given to those with previous exposure to quantum computing. Unlike other traditional science areas, quantum computing is not typically taught at the undergraduate level; it is very interdisciplinary, incorporating physics, computer science, and math. Therefore, it’s harder for students to have the opportunity to be exposed to quantum computing. This is one of the biggest challenges to building a quantum workforce.
“This is what makes our summer school so crucial. Traditional university majors do not often emphasize interdisciplinary topics like quantum computing, and we are filling that gap,” Coles said.
The summer school students will be part of that new quantum workforce, helping to create the foundations for a revolutionary type of computing.
“Quantum computing is still new, but it has a unique and important role to play in the future and in national security,” Coffrin said.
The students may be young, but their projects and contributions are all very demonstrable. Many students continue working with their Los Alamos mentors after the completion of the summer school.
“It is very exciting to be working on near-term quantum algorithms. I feel how I imagine computer scientists in the 1950s and 1960s felt when they were creating the first algorithms to be run on the earliest classical computers,” Beckey said. “We are in the early stages of an exciting new computing paradigm, and LANL is the place to be if you want to be surrounded by leaders in the field.”
Funding and mission
Quantum Summer School is funded by the Information Science and Technology Institute (ISTI). It supports the Laboratory’s Global Security mission area and the Integrating Information, Science, and Technology for Prediction capability pillar.
Technical contacts: Lukasz Cincio, Patrick Coles, and Carleton Coffrin
High Performance Computing
LANL partners with Eideticom to bring computational storage processor to the market
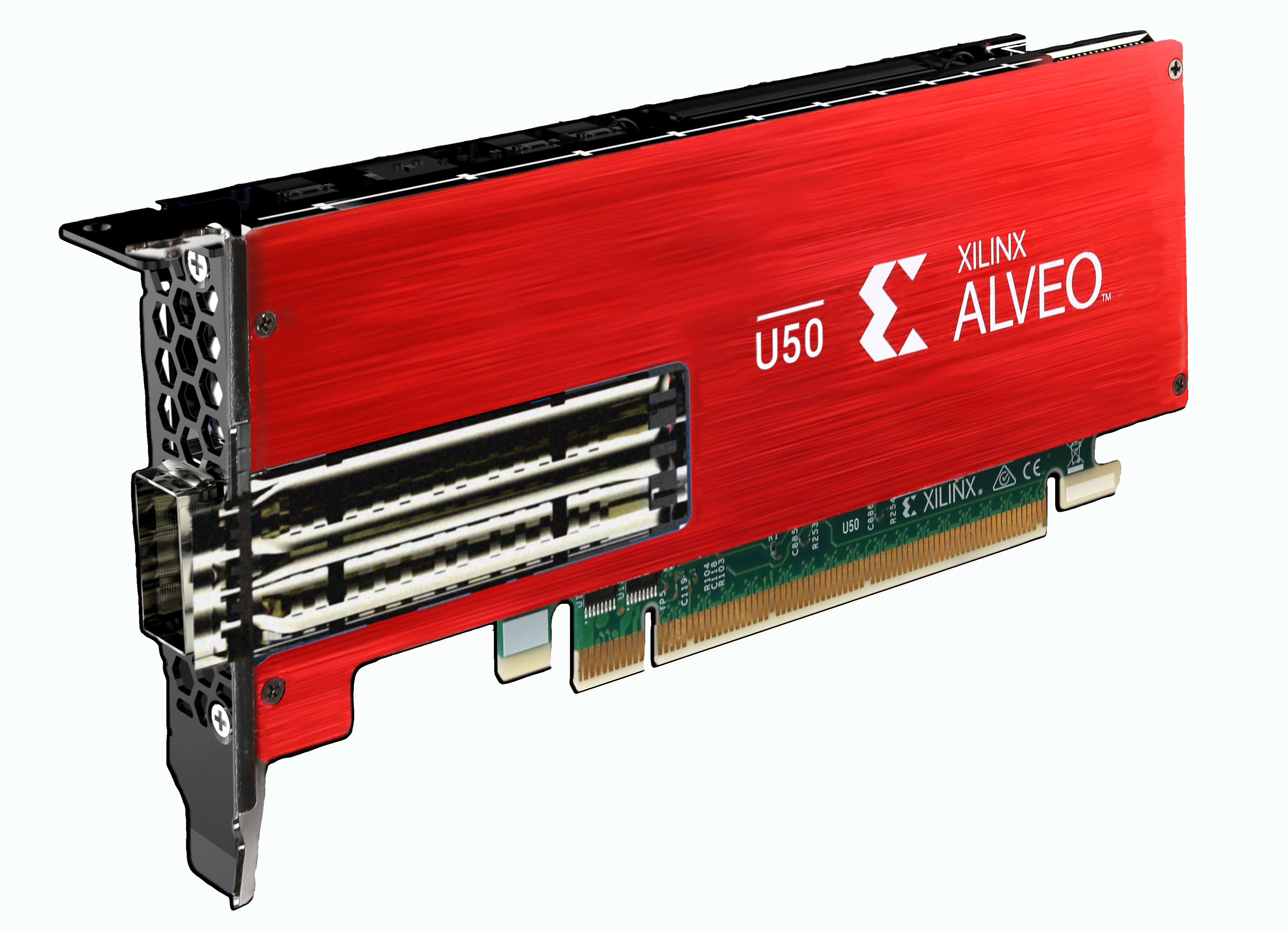
One of the NoLoad® computational storage system models available through Eideticom.
Researchers in High Performance Computing partnered with Eideticom, a world-class computational storage solution company for cloud and enterprise data centers, to bring a novel solution to the computational storage market. NoLoad® is a small piece of hardware that offers data compression and accelerated data storage.
“Applications using sophisticated storage systems often perform far below the capabilities of the underlying storage media and networking hardware, limiting the performance of large-scale data-driven scientific discovery,” said Brad Settlemyer, HPC Design. “NoLoad® CSP is a powerful solution because it embeds processing power directly into the storage fabric, which dramatically increases performance, efficiency, and flexibility while lowering costs.”
Watch a video on the NoLoad® CSP technology.
NoLoad Benefits
Previously, storage architects were forced to choose between fast compression algorithms that achieve poor compression rates but don’t excessively tax the memory subsystem or more effective multi-pass compression algorithms that are unable to match the performance of modern, fast solid-state disks. Compression quality is especially important for the massive, hard-to-compress data sets generated and analyzed by the extreme-scale computing systems used at Los Alamos to perform simulation science. NoLoad® CSP eliminates the tradeoff between compression performance and quality by leveraging a data parallel accelerator able to provide both high levels of throughput and excellent data compression. It is truly a market disrupting product.
In terms of numbers, NoLoad® CSP stores 26 percent more data onto a storage device while simultaneously achieving 8 percent greater throughput. It’s important to note that this was measured using the most challenging data sets in Los Alamos’ data center. Using more common data sets, the NoLoad® CSP is able to write 300 percent more data to a storage device with a greater than 200 percent throughput increase. This amazing data reduction and performance is achieved while using 70 percent less CPU power.
In use at LANL
Los Alamos is currently using NoLoad® CSP inside of real-production parallel file systems. The data set reduction and performance improvements are seamlessly available to users, without the need for changing code. The file system transparently compresses and decompresses the data.
The NoLoad® device is embedded along the storage system data path, so the compression algorithm computes as the data streams from the processor into the storage device. The researchers illustrated the ease with which the device can be programmed to support scientific data.
However, NoLoad® can do more than just data compression; it can also be programmed to perform erasure coding, data analytics, and machine learning classification. Partnering with industry leaders on emerging technologies, such as the Los Alamos partnership with Eideticom in developing the first standards-compliant computational storage processor, is critical to maintaining technology leadership for our national laboratories and supporting Los Alamos’ national security mission.
“Deploying relevant, advanced technology for use by our scientists is critical to enabling the data analysis that ensures the U.S. nuclear stockpile is both safe and reliable,” Settlemyer said.
Funding and mission
NoLoad® and the collaboration between Eideticom and Los Alamos National Laboratory were funded by the Advanced Simulation and Computing (ASC) Program as part of the Computational Systems and Software Environments (CSSE) program element. This work supports the Laboratory’s Nuclear Deterrence mission area as well as the Integrating Information, Science, and Technology for Prediction capability pillar.
Available for purchase
NoLoad® CSP is available for purchase through Eideticom. The price of the product varies depending on licensing terms, but evaluation licenses are available for as little as $1,000. This is a very low procurement cost for high-quality, rapid data compression.
NoLoad® CSP full team from Los Alamos: Brad Settlemyer, Dominic Manno, Brian Atkinson, and Gary Grider.
Related links: Eideticom https://www.eideticom.com/
Technical contact: Brad Settlemyer
Infrastructure Enhancement
3D model will enable remote design for new Crossroads supercomputer
The Los Alamos Advanced Simulation and Computing Program is readying its infrastructure for a new flagship supercomputer, Crossroads, which will be located in the secure Strategic Computing Complex. But the COVID-19 pandemic and the subsequent need for remote work has created challenges, rendering much in-person work impossible.
To remedy this issue, Los Alamos engineers developed a 3D Building Information Model (BIM) specifically for the design and installation of Crossroads. The BIM uses light detection and ranging (LIDAR) scans together with global positioning systems (GPS) to create highly accurate building layouts.
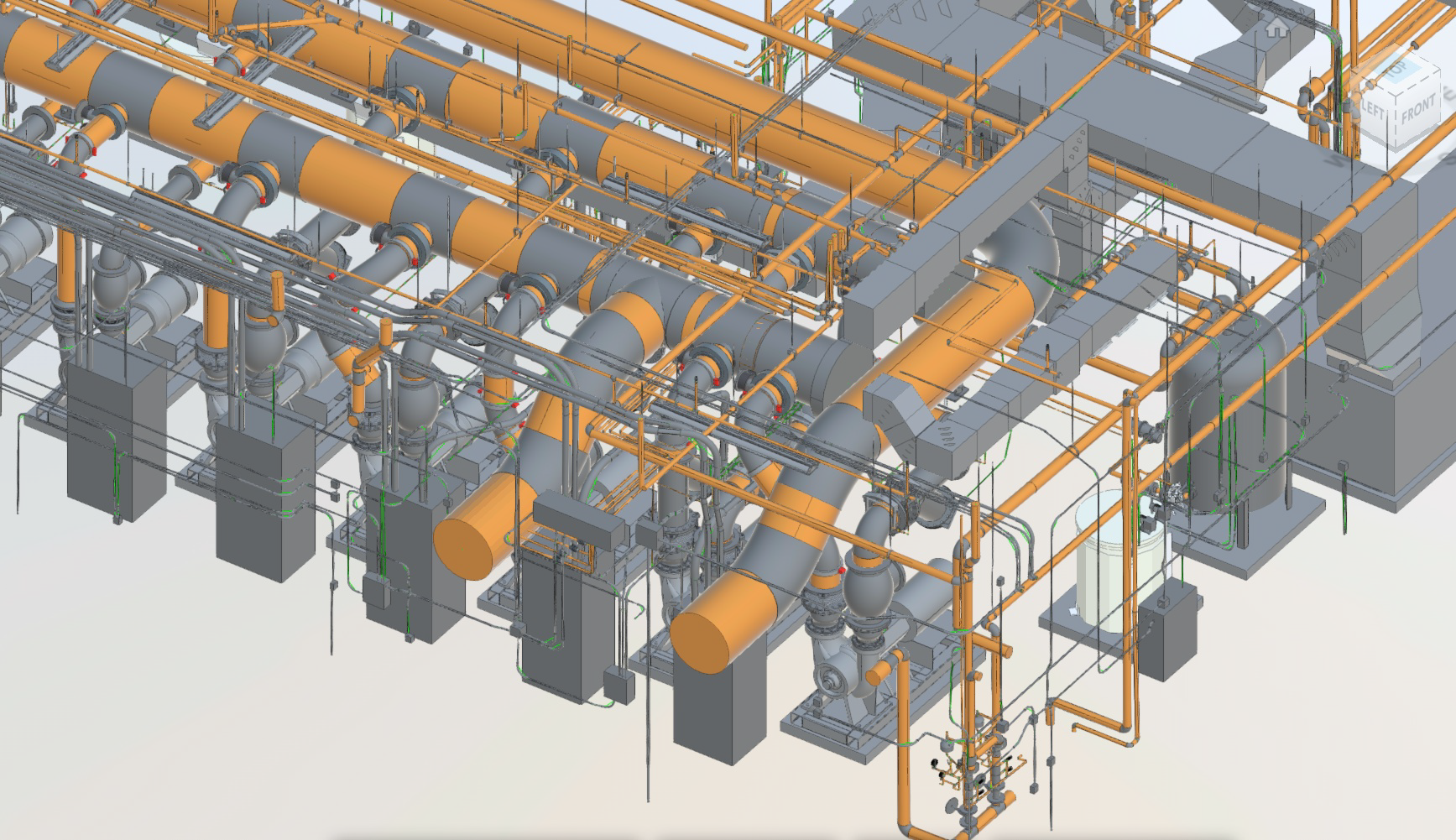
An example of the 3D model and the interdisciplinary design it portrays.
“The challenge was in converting the LIDAR scan into a usable computer-aided design and BIM model,” said Matt Brazil, Engineering Project Delivery lead (ES-EPD) for High Performance Computing engineering. “This requires the designer/drafter and scanner to understand the LIDAR scan and identify the system, structure, or component within the scan.”
The model provides a highly accurate 3D spatial rendering that allows architectural and engineering (A/E) firms to efficiently collaborate and coordinate on interdisciplinary designs: mechanical, electrical, plumbing, etc. Without traveling to Los Alamos and to a secure facility, these A/E associates can make the needed spatial measurements and designs to keep the Crossroads installation on track—with the goal of having Crossroads installed by late 2021. Crossroads will include a number of major advances in computing technologies for simulating very complex physical systems.
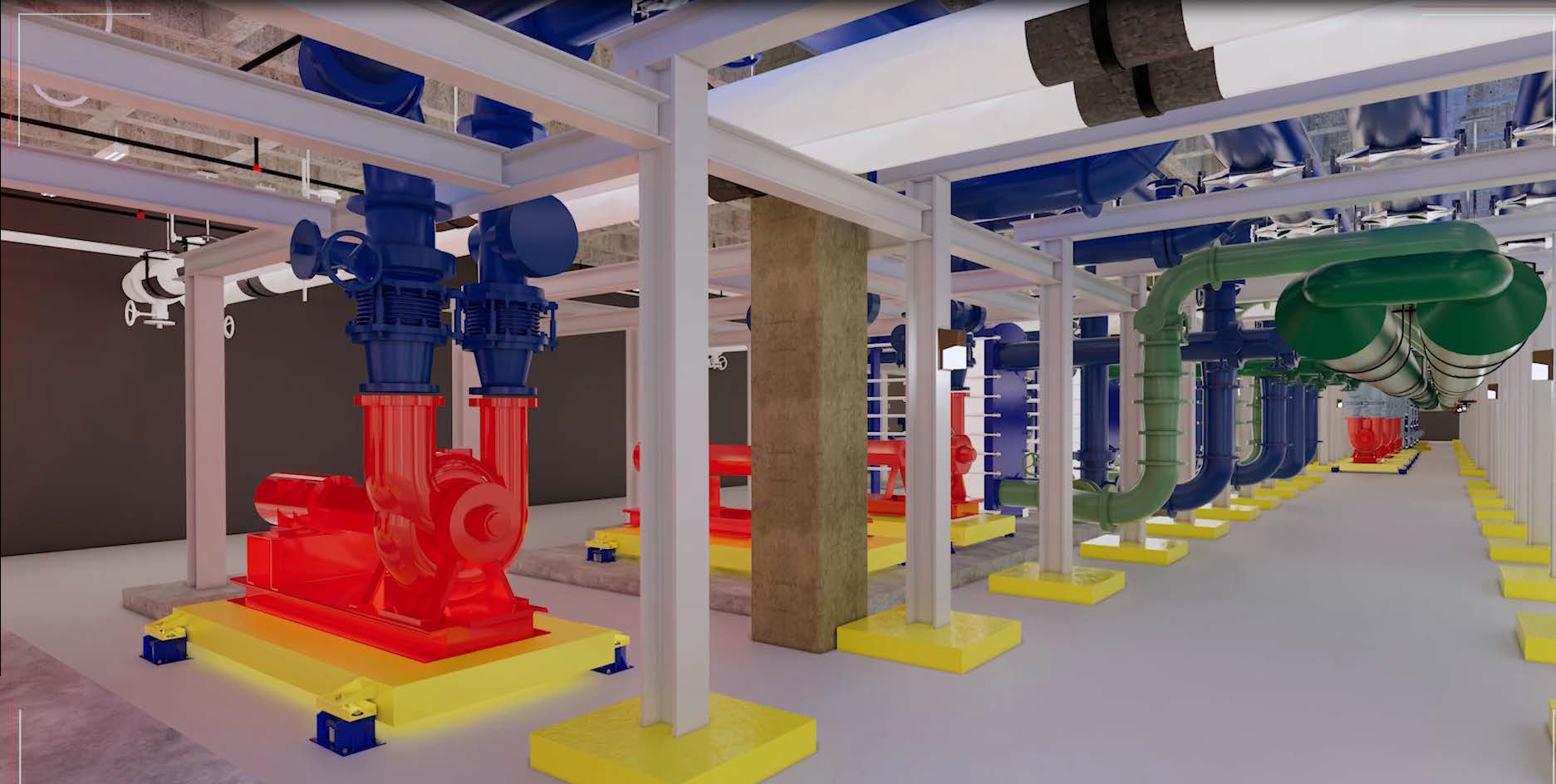
The BIM enables highly accurate measurements without the need for physical contact.
Additionally, BIM can be used by maintenance and operations. This is achieved by providing the model with technical and preventative maintenance plan data for modeled equipment and components.
“This allows a BIM user, such as a facility operator or facility engineer, to click on a piece of equipment within the model and know everything about that piece equipment, without having to leave their desk or go into the field,” Brazil said.
Beyond Crossroads, BIM will become the as-built model of the Strategic Computing Complex. This enables any A/E firm to collaborate on a model that can provide the design for new infrastructure upgrades at the facility. It is the intent that all future infrastructure projects will take this approach.
Funding and mission
The 3D BIM was funded by the Advanced Simulation and Computing Program. The work supports the Laboratory’s Nuclear Deterrence mission area and the Complex Natural and Engineered Systems capability pillar.
Materials Physics and Applications
Experimental demonstration of advanced photonic systems leads to boosted performance
Researchers from Los Alamos National Laboratory, in collaboration with Duke University, demonstrated that ultrathin artificial composites, called metasurfaces, help realize unprecedented electromagnetic phenomena.
In general, the fundamental principle of Lorentz reciprocity governs the functionalities of photonic systems, imposing constraints such as re-emission of absorbed solar energy from photovoltaic cells or transmitting antennas listening to their echoes. This unwanted reciprocity can result in decreased performance.
To boost the performance of these photonic systems, the researchers aimed to break the reciprocity.
“Our goal in this paper was to demonstrate the complete violation of Lorentz reciprocity via mode-conversion,” said Abul Azad, Center for Integrated Nanotechnologies (MPA-CINT).
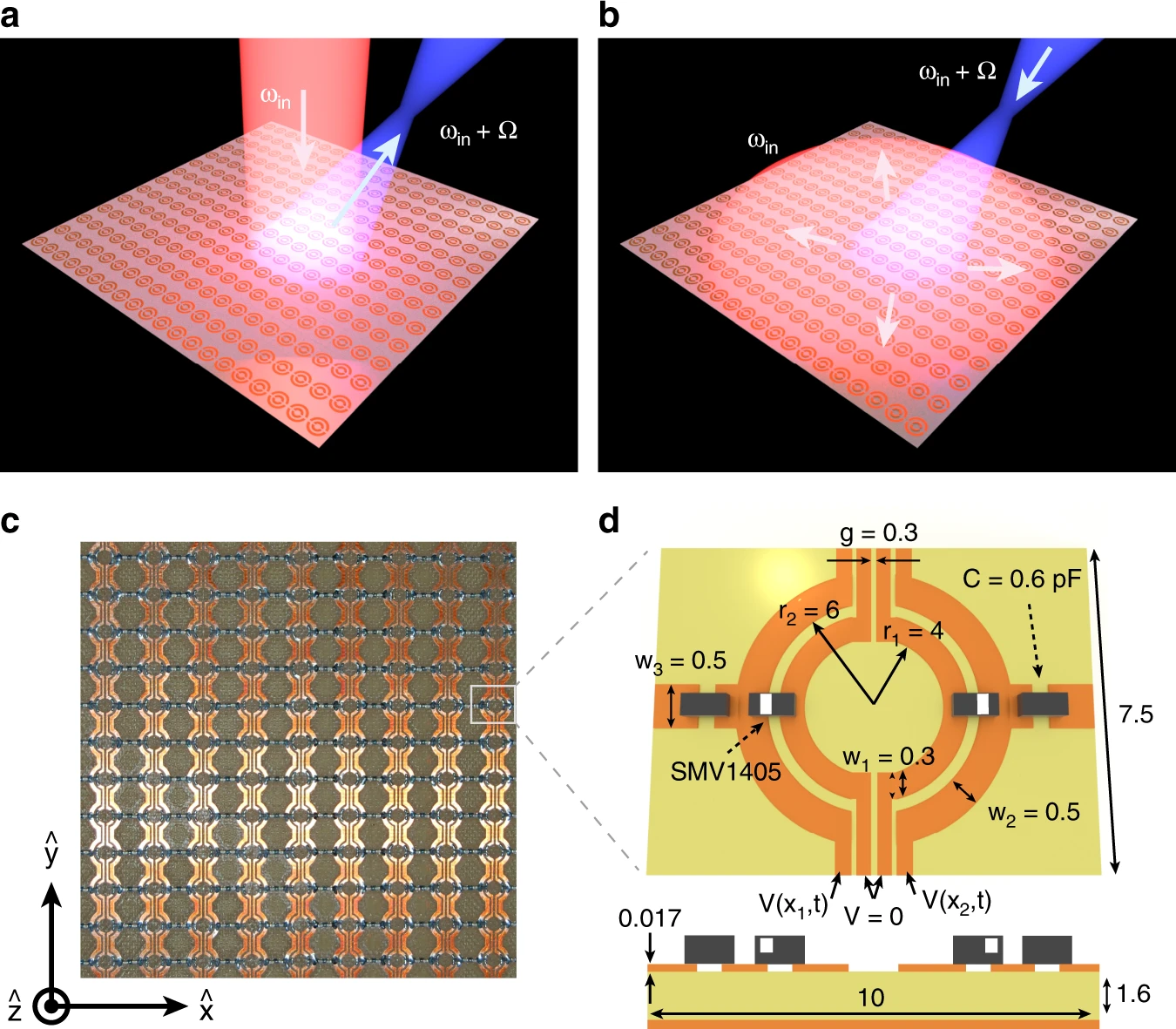
(a) An incident beam impinging on the metasurface is converted into a different frequency harmonic that can be focused at any desired focal point. (b) Breakdown of Lorentz reciprocity can be shown by probing the time-reversed process. (c) Photograph of the spatio-temporally modulated metasurface. (d) Top view and cross-section of the unit cell.
Violating the Lorentz theorem
Elimination of unwanted reciprocity in photonic systems is challenging. To do so requires violating the Lorentz reciprocity theorem, which states that in a linear, time-independent system with symmetric constitutive optical tensors, the ratio between received and transmitted fields are the same for forward and time-reversed propagation directions.
The researchers introduced a spatio-temporally modulated metasurface (STMM)—an artificial composite material that can convert an incident light beam into a different (non-reciprocal) wave harmonic. In the presence of spatio-temporal modulation, the metasurface allows reflection for the forward scattering direction via photon-to-photon conversion. However, for backward scattering, photons completely couple to surface waves via photon-to-surface wave conversion. This introduces an extreme breakdown of Lorentz reciprocity, leading to giant optical isolation, as no propagative modes are radiated in reverse scattering.
Efficiencies can be improved
The researchers focused on making theoretical predictions and confirming those predictions through experiments. Their work sets the foundation for further modification and improvement.
One such future improvement for the STMM is increasing the conversion efficiency. Currently, most of the reflected power resides in the fundamental harmonic. This can be addressed by using non-harmonic asymmetric time-modulation protocols. In principle, these modifications could result in conversion efficiencies greater than 80 percent.
By independently addressing each individual resonator, the STMM can be used for three-dimensional wave-front shaping, including dynamic beam steering, focusing, and generation of optical angular momentum. The STMM will enable compact multifunctional photonic components with built-in isolators for wireless communication and remote sensing.
Funding and mission
This research was supported by a LANL Laboratory Directed Research and Development (LDRD) award. Part of this work was performed at the Center for Integrated Nanotechnologies (CINT), a U.S. Department of Energy, Office of Basic Energy Sciences user facility. The work supports the Laboratory’s Energy Security mission and the Materials for the Future capability pillar.
Reference: Cardin, A. E., Silva, S. R., Vardeny, S. R. et al. “Surface-wave-assisted nonreciprocity in spatio-temporally modulated metasurfaces.” Nat Commun 11, 1469 (2020). https://doi.org/10.1038/s41467-020-15273-1
Technical contact: Abul Azad
X-Computational Physics
New record for highest pressures ever achieved in laboratory equation-of-state measurements
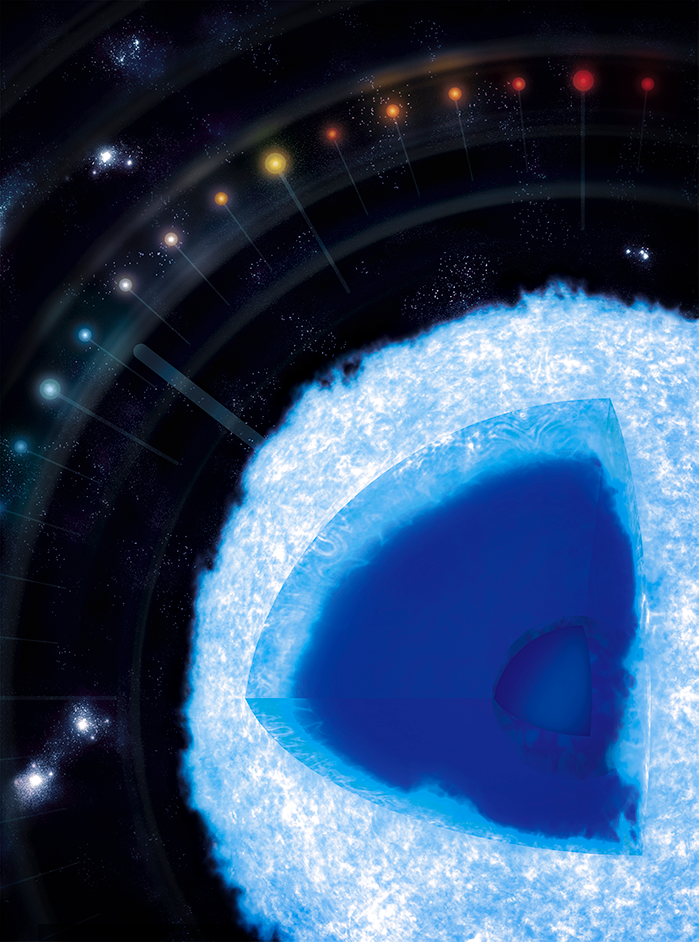
Artist concept showing the inner structure of a white dwarf star with a solid crystallized core of carbon and oxygen surrounded by a dense plasma envelope. The outer part of the star is constantly churning by convection. This is the region probed by the new experiment. White dwarfs can be used as cosmic clocks in a form of stellar archeology, called cosmochronology, to uncover the age and the history of our galaxy, the Milky Way. Image courtesy of Stanford Linear Accelerator (SLAC).
A new experimental record was set by researchers at Lawrence Livermore National Laboratory’s National Ignition Facility (NIF) – the world’s highest-energy laser system – and collaborators, including Los Alamos researcher Didier Saumon of Materials and Physical Data (XCP-5). The researchers measured the equation of state (EOS) on the shock Hugoniot at pressures exceeding 300 Mbar. Before this, no one had achieved such measurements beyond 60 Mbar. Their results were published in Nature.
Using a plastic target made of carbon and hydrogen in a 50-50 ratio, the researchers were able to achieve the stripping of all six carbon valence electrons under shock conditions.
“This is the first time conditions matching those inside a white dwarf star, with extreme pressure, can be replicated in a lab to measure the properties of carbon,” Saumon said.
Understanding and measuring matter in these extreme environments is essential for a number of fields: the Laboratory’s mission and validating Lab models, inertial confinement fusion (ICF) and bringing the concept of practically limitless clean energy to fruition, and unraveling the history of the Milky Way with white dwarf stars.
A new technique at NIF
It was Livermore collaborators who developed a new technique at NIF that allowed for equation-of-state measurements at these extreme pressures in a laboratory for the first time. For their hydrocarbon target, the pressures reached 450 Mbar, or about 120 times the pressure at the center of the Earth. To give perspective, 1 bar equals 1 atmosphere of pressure, and 1 Mbar equals 1 million atmospheres of pressure. The researchers anticipate being able to reach as much as 1,000 Mbar in the near future.
In their current experiment, a solid plastic target was used, but the technique can be applied to a variety of materials, such as those used in inertial confinement fusion research.
Relating to white dwarf stars
It was Saumon who made the connection between the current experimental conditions and white dwarf stars. The data from these lab experiments will help improve white dwarf star models and ultimately our estimate of the age and history of the Milky Way.
White dwarfs are dead stars; they no longer have an energy source because the nuclear fusion has stopped. They are initially hot and gradually cool, which enables scientists to estimate their age from their temperature.
“This research is really the beginning,” Saumon said. “It opens so many possibilities for advances in astrophysics, ICF, and dense plasma physics.”
Funding and mission
The work was supported by a Laboratory Directed Research and Development award, the University of California, the NNSA, and the DOE Office of Science. The NIF Discovery Science Program provided access to their facility. The research supports the Laboratory’s Global Security mission area and the Complex Natural and Engineered Systems capability pillar.
List of collaborating institutions: Lawrence Livermore National Lab, University of Rochester, General Atomics, Université de Montréal, University of Notre Dame, Helmholtz-Zentrum Dresden-Rossendorf, Technische Universität Dresden, GSI Helmholtzzentrum für Schwerionenforschung GmbH, UC Berkeley, SLAC National Accelerator.
Reference: Kritcher, A.L., Swift, D.C., Döppner, T. et al. A measurement of the equation of state of carbon envelopes of white dwarfs. Nature 584, 51–54 (2020). https://doi.org/10.1038/s41586-020-2535-y
Technical contact: Didier Saumon