STE Highlights, October 2020
Awards and Recognition
Earth and Environmental Sciences
Improving climate system understanding through submicron aerosol data
Materials Science and Technology
Rare experiment leads to discovery of new material defect mechanism
Foundational research for emerging class of materials: oxysulfide perovskites
Physics
Los Alamos scientists author key chapters for Deep Underground Neutrino Experiment design
ICF advancement: First observation of scale invariance in the high-energy-density regime
Awards and Recognition
LANL-developed surfactants win two technology awards
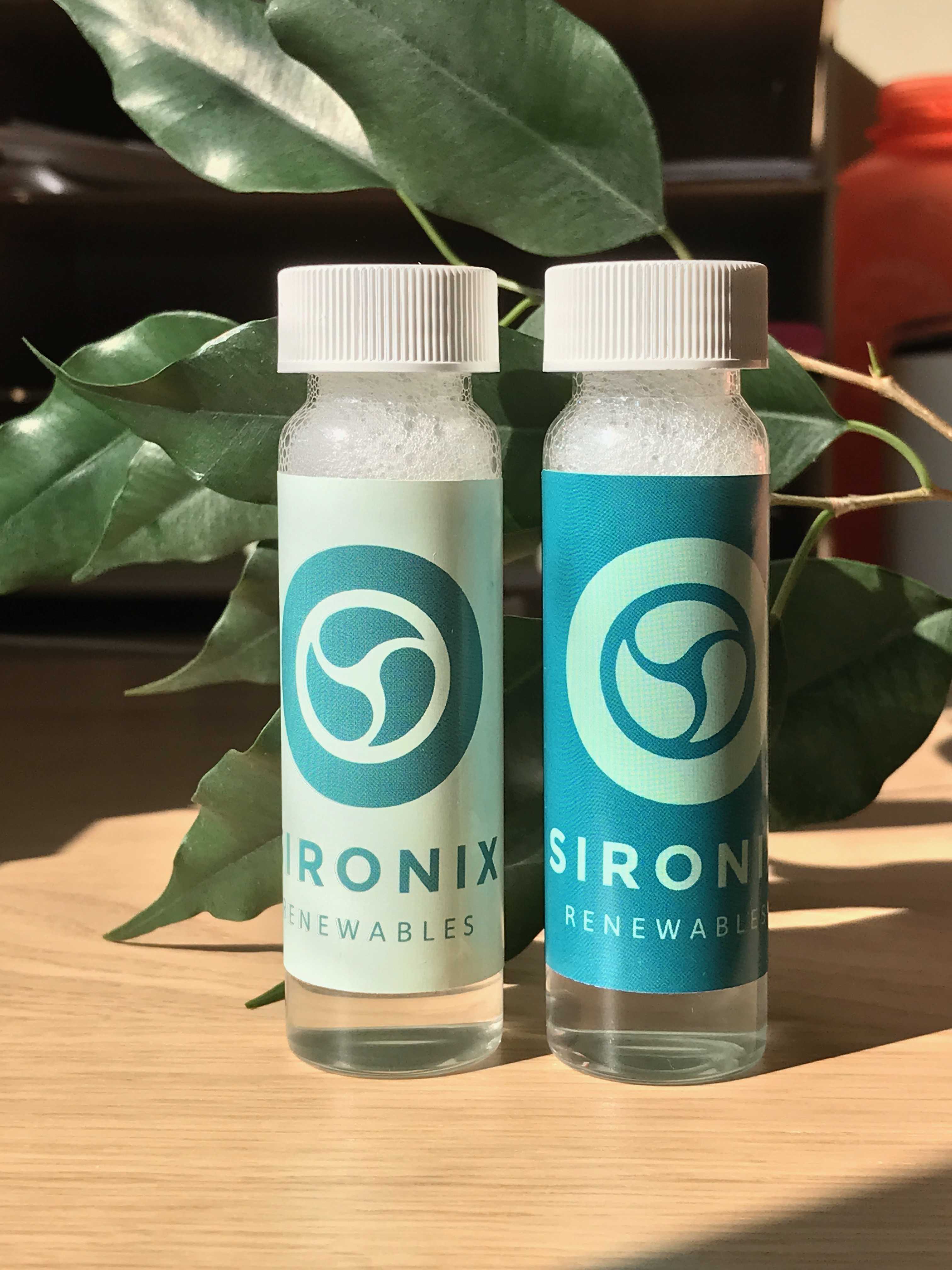
A new type of surfactant developed by a Los Alamos team with Sironix Renewables
Los Alamos chemistry researchers, led by Andrew Sutton of Inorganic Isotope & Actinide Chemistry (C-IIAC) partnered with Sironix Renewables to create a new type of surfactant—one that is better for the environment. Surfactants are the key ingredient in laundry detergents, but they are also used in cosmetics, paints, coatings, and much more. Sutton and his team created a new class of surfactant derived from sustainable biomass. It is the first non-toxic, non-irritating surfactant to work effectively in cold or hard water.
The new technology garnered an R&D 100 Bronze Medal in Green Technology as well as a Mid-Continent Notable Technology Award from the Federal Laboratory Consortium. This technology, along with the partnership it spurred between LANL and industry, is a prime example of successful technology transfer. With help from the Los Alamos Feynman Center, the synthesis process was patented, partnered, and transferred to accelerate this technology out of a federal lab and into the marketplace.
The new surfactants are currently being tested by large, brand-name companies for incorporation into their shelf-ready products.
Bioscience
First epigenetic manipulation of biofuel algae boosts performance factors
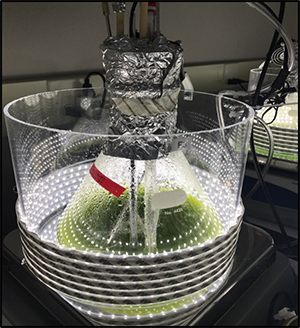
Picochlorum soloecismus cultivation
A cutting-edge experiment led by Los Alamos bioscientists reported the first-ever epigenetic manipulation of the biofuel platform species Picochlorum soloecismus.
Rather than directly altering the DNA of the algae used for biofuel, which requires significant time and effort, the researchers purposefully modified the markings on top of the DNA: the epigenetics. These epigenetic marks can be inherited, and understanding the inheritance of these marks and their role in gene expression (turning genes on and off) has been lacking in algae. This experiment provides the first look at epigenetic marks in P. soloecismus and evidence for performance benefits to P. soloecismus via epigenetic manipulation.
“Epigenetic manipulation offers a non-GMO strategy, whereby we alter the environment of the microalgae (by adding a chemical). The change in the environment causes the algae to change its behavior,” said Christina Steadman, Bioenergy and Biome Sciences (B-11). “In a sense, we just give the algae a little push toward the behavior we want – to make more biofuel precursors – rather than fundamentally altering what they are.”
Steadman relates this to the real life example of a person training at altitude to enhance their performance on the slopes: the training impacts their physiology but doesn’t fundamentally change who they are (the DNA). Likewise, the mechanism by which the algae’s behavior and physiology change is through epigenetic marks, not genetic engineering.
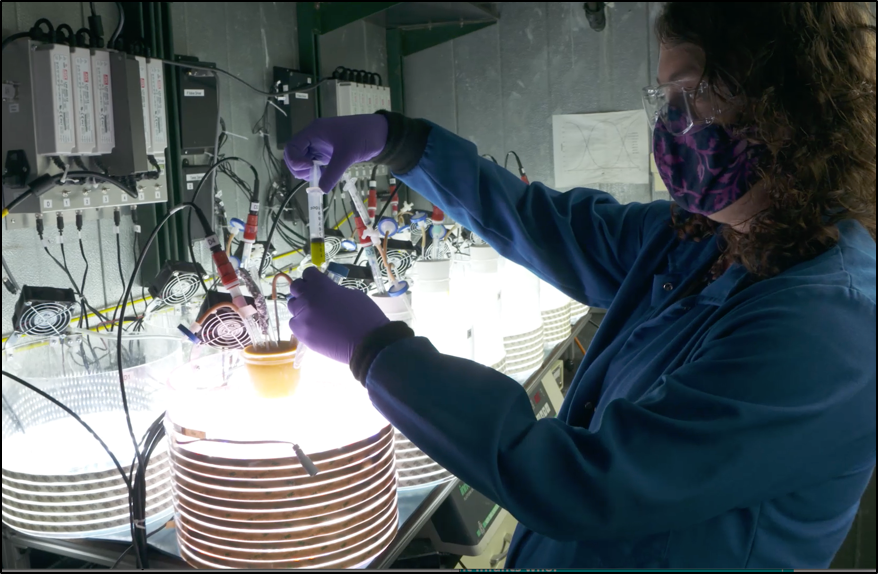
Christina R. Steadman demonstrates the process of epigenetic manipulation of algae.
Methylation as a marker
DNA is specifically marked with methyl groups, indicating where DNA should and should not be transcribed. The researchers noticed that this marking could be altered during the algae growth phase through nitrogen starvation and 5AZA (a DNA methyltransferase inhibitor) treatment—both environmental factors.
The altered marking led to increased lipid production and cell size, which are considered positive performance factors for biofuel and biofuel products.
“Genes involved in several biological processes, including fatty acid synthesis, had altered methylation ratios; we hypothesize these changes are potentially responsible for the phenotype of early induction of carbon storage as lipids,” Steadman said.
Making affordable, clean fuel
This experiment is foundational to the epigenetic manipulation technique of fine-tuning algae growth, metabolism, and environmental adaptation. Inexpensive and effective production of biofuel is in high demand, and LANL scientists are currently applying this technique to other algae types that are also suitable for biofuel production.
Global demand for energy is projected to grow by about 25 percent through 2040. To expand supplies—while increasing efficiency and mitigating emissions—biofuel will be an important alternative to petroleum-derived fuels.
Funding and mission
This work was supported by the DOE Office of Energy Efficiency and Renewable Energy under contract from the Bioenergy Technologies Office. The research supports the Laboratory’s Energy Security mission area and the Science of Signatures capability pillar.
Reference: Christina R. Steadman, Shounak Banerjee, Yuliya A. Kunde, Claire K. Sanders, Babetta L. Marrone and Scott N. Twary. “Inhibition of DNA Methylation in Picochlorum soloecismus Alters Algae Productivity.” Front. Genet. 11:560444. doi: 10.3389/fgene.2020.560444and Biome Sciences
Technical contact: Christina R. Steadman
Chemistry
Novel technique leads to record-high quantum dot doping
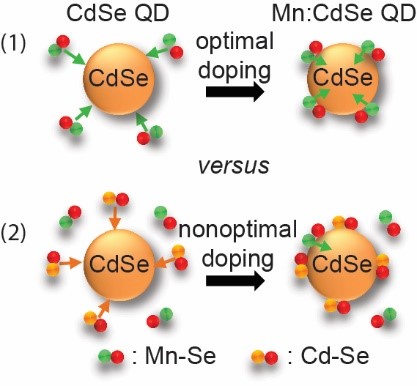
During “optimal” doping (1), incorporation of Mn occurs solely via addition of Mn-Se units, while in the “nonoptimal” case (2), it competes with unwanted addition of Cd-Se units.
The Journal of the American Chemical Society recently published a cover feature highlighting a Los Alamos-developed synthesis technique stemming from a two-decade collaboration between LANL Chemistry (C) division and Materials Physics Applications (MPA) Division scientists.
Together, the scientists achieved record-high manganese doping (>30% of all cations) of quantum dots, which makes them more effective for sunlight harvesting, electron photoemission, and advanced imaging.
“We need high-quality manganese-doped quantum dots for multiple projects dealing with both fundamental and applied aspects of spin-exchange interactions between magnetic impurities and intrinsic quantum dot states,” says Victor Klimov of Physical Chemistry & Applied Spectroscopy (C-PCS), lead author. “These include our work on manipulation of ultrafast hot-electron dynamics for advanced photoconversion, the studies of photon down-conversion for applications in luminescent solar concentrators, as well as our research into novel hybrid states of matter, such as exciton magnetic polarons. All of these projects will greatly benefit from the availability of quantum dots with precisely controlled contents of manganese impurities.”
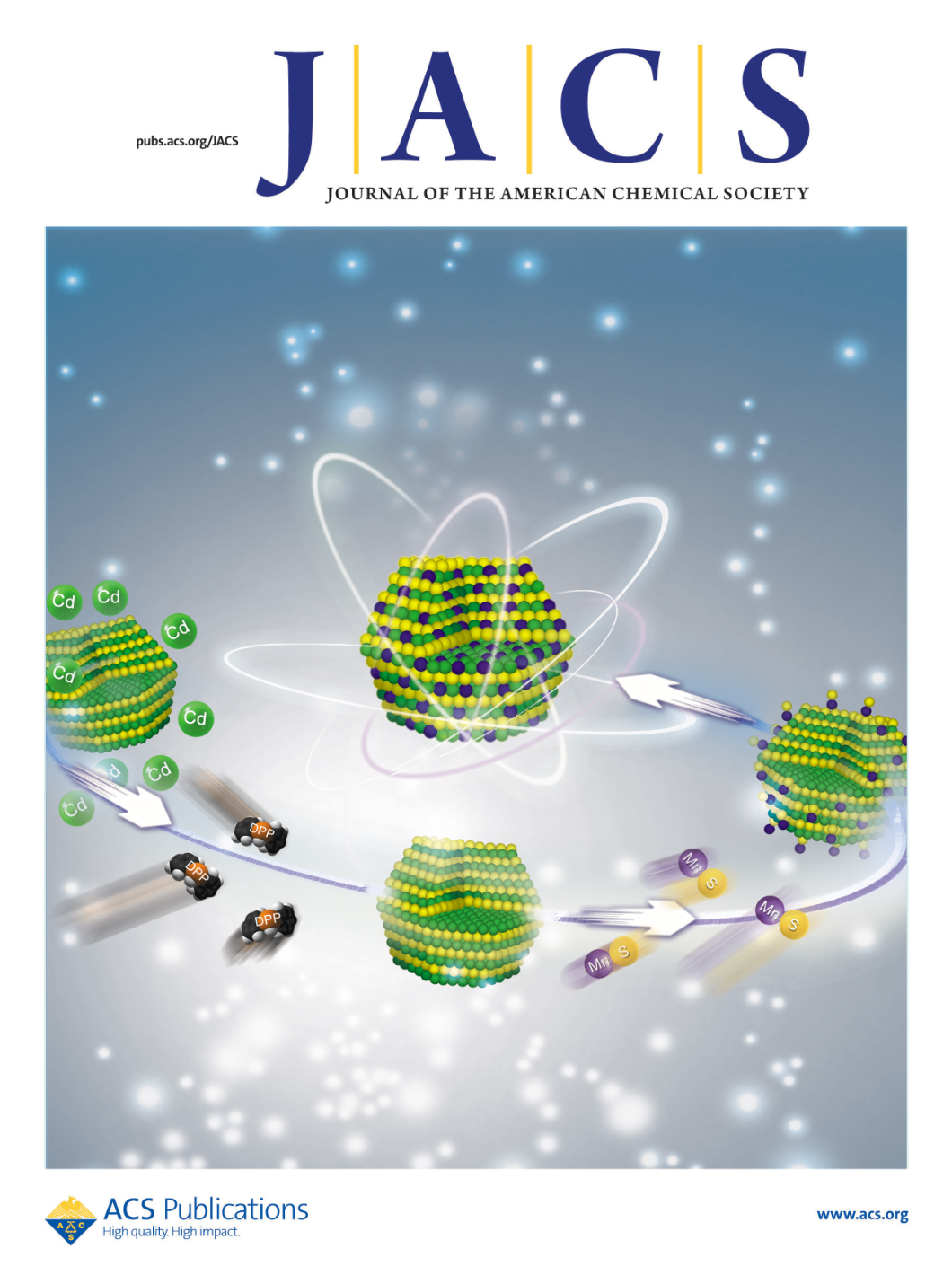
Doping of CdSe quantum dots with manganese enacts novel functionalities exploited in color conversion, sunlight harvesting, electron photoemission, and advanced imaging. By introducing functional secondary phosphine impurities into quantum dot synthesis, Los Alamos researchers achieve a virtually ideal regime of doping which proceeds solely via incorporation of Mn without unwanted side reactions. Cover graphic design by Jun Du and Ho Jin.
Uniform doping is key
The synthesis outlined in this research resolves major problems of previous doping techniques, which included poor reproducibility, increased quantum dot polydispersity, and very slow dopant incorporation. Essentially, the amount of manganese and how that manganese was distributed throughout the quantum dots was not controlled to the degree that is needed.
The researchers attributed these issues to unreacted cadmium in the samples of original quantum dots used in the doping reaction. In particular, the presence of remnant cadmium stimulates unwanted quantum dot post-growth via addition of Cd-Se units, which competes with the desired incorporation of Mn-Se species. Further, they noticed that the amount of unreacted cadmium decreases with increasing abundance of unintentional secondary phosphine impurities that occur in typically tertiary phosphine ligands.
Cleverly, the researchers harnessed those impurities by modifying the quantum dot synthesis to include controlled amounts of secondary phosphine species in the form of diphenylphosphine. These “functional” impurities improved consumption of cadmium precursors and thereby aided uniform manganese incorporation without unwanted side reactions.
Magnetic Field Lab confirms results
The manganese is paramagnetic (spin 5/2) and, therefore, detectable using LANL’s National High Magnetic Field Lab. Optical measurements in strong applied magnetic fields were performed on the magnetically-doped quantum dots to quantify the exact amount of incorporated manganese.
The amount of magnetic material incorporated into the quantum dots was determined by measuring the difference in quantum dot optical absorption for right- and left-circularly-polarized light, as a function of applied magnetic field.
“Our high magnetic system very nicely complements many of the spectroscopy capabilities in the LANL chemistry labs, providing a platform to perform spectroscopy in both large magnetic fields (to 7 tesla) and very low temperatures (to 1.5 Kelvin),” said Scott Crooker (MPA-MAG).
The incorporation of magnetic manganese ions into semiconductor quantum dots leads to enhanced magneto-optical properties. With this high level of doping achieved, antiferromagnetic coupling between neighboring manganese spins becomes apparent in the magnetic and magneto-optical response. The observation of the onset of antiferromagnetic effects helped optimize the level of doping by maximizing the number of “free” isolated manganese spins.
Although the high and uniform manganese doping is significant in and of itself, the identification of the issue—unreacted cadmium—is of considerable importance to other areas of quantum dot chemistry, such as cation exchange reactions, controlled surface modifications, and growth of complex heterostructures.
Funding and mission
Funding for the studies came from the Solar Photochemistry Program of the Chemical Sciences, Biosciences and Geosciences Division, Office of Basic Energy Sciences, Office of Science, U.S. Department of Energy. The characterization of magnetooptical properties of Mn-doped quantum dots work was supported by the Los Alamos Laboratory Directed Research and Development (LDRD) program. The work supports the Laboratory’s Energy Security mission area and the Materials for the Future capability pillar.
Reference: Ho Jin, Mateusz Goryca, Michael T. Janicke, Scott A. Crooker, and Victor I. Klimov. “Exploiting Functional Impurities for Fast and Efficient Incorporation of Manganese into Quantum Dots.” J. Am. Chem. Soc. 2020. Cover article, JACS spotlight https://pubs-acs-org.lanl.idm.oclc.org/doi/10.1021/jacs.0c08510
Technical contact: Victor I. Klimov
Earth and Environmental Sciences
Improving climate system understanding through submicron aerosol data
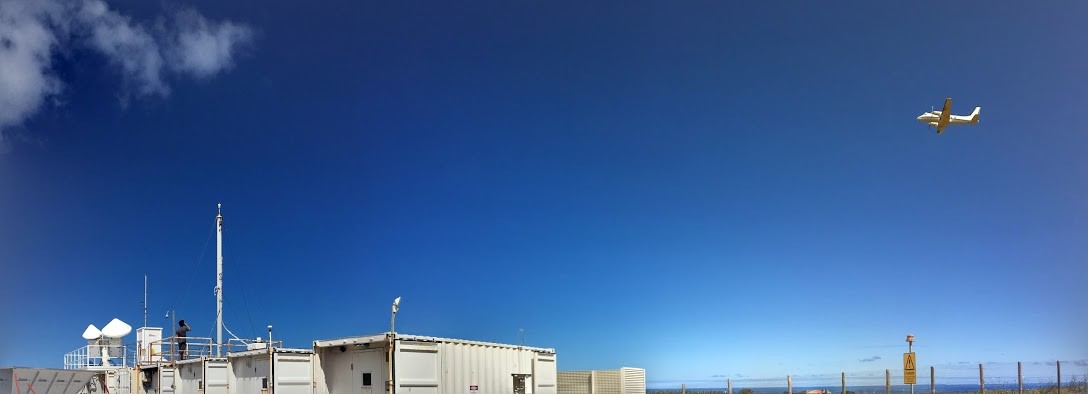
A DOE ARM Aerial Facility research plane flies over the eastern North Atlantic aerosol and cloud experiments facility.
A research team led by scientists in the Earth and Environmental Sciences (EES) Division made key contributions to improving climate modeling and predictions by reducing a well-known uncertainty in aerosol–cloud interactions and aerosol processes.
The researchers noted that regions dominated by clean atmospheric conditions are the most susceptible to aerosol perturbations, and so the scientists decided to investigate in the eastern North Atlantic Ocean from Graciosa Island.
“The remote marine environment with low-lying clouds in the Azores has one of the largest uncertainties in climate models,” said Francesca Gallo, Earth System Observations (EES-14).
Data were collected at the U.S. Department of Energy's Atmospheric Radiation Measurement (ARM) user facility located on the island. For the experiments, a supplemental facility was deployed by Allison Aiken (EES-14) with support from LANL’s Field Instrument Deployments & Operations (FIDO) team; this was in addition to the Aerosol Observing System (AOS) at the central facility. Through this process, new data products were developed and can be found online via the ARM Data Discovery tool.
![Daily average (a) summer and (b) winter total submicron [N(tot); < 1 micron diameter] and accumulation mode [N(Ac); 100–300 nm diameter] aerosol number concentrations. Original Aerosol Observing System data in black and the new data product with local aerosol events removed in green, with percent reductions in blue.](_images/2020-10/ees-fullsize-aerosal2.jpg)
Daily average (a) summer and (b) winter total submicron [N(tot); < 1 micron diameter] and accumulation mode [N(Ac); 100–300 nm diameter] aerosol number concentrations. Original Aerosol Observing System data in black and the new data product with local aerosol events removed in green, with percent reductions in blue.
Particle size and season are factors
Submicron aerosol concentrations and size distributions were used along with collocated meteorological data (e.g., wind direction) to associate high-concentration aerosol events with potential local aerosol sources. Data was collected both from the ground-based facilities and from research flights.
Aitken-mode (< 100 nm diameter) and accumulation-mode (100–300 nm) aerosols were impacted differently.
“Accumulation-mode aerosols form cloud droplets and were less impacted than Aitken-mode aerosols, which are associated with new particle formation and growth, especially in the summer,” Aiken said.
The researchers used the analyses to generate a regional aerosol baseline, which they could then extract from data sets to reduce uncertainty. Often, for regional aerosols, there are short time periods impacted by local sources that do not represent larger regional processes. Therefore, time resolution is an important factor. The system collected numerous aerosol properties at time resolutions on the order of minutes to seconds — real-time data taken right at the measurement site.
After removing the local aerosol event periods, monthly submicron aerosol mean number concentrations were reduced by factors of 1.65 in the summer and 1.55 in the winter.
Applying this method elsewhere
The researchers developed a numerical “novel aerosol mask,” which elucidates the regionally representative submicron aerosol number concentrations near the ground and in the column during flights. A mathematical algorithm was developed based on these considerations, observational data, and the season.
This method can be applied elsewhere to reduce uncertainty in climate models that force data to make predictions or use observational data for model validation. Aerosols play a key role in cloud formation, precipitation, and solar radiation. The research will improve data quality for radiative forcing calculations, such as the aerosol single scattering albedo, asymmetry parameter, mass scattering efficiency, mass absorption coefficient, and hygroscopic growth.
Funding and Mission
This work was supported by the Department of Energy Atmospheric Radiation Measurement (ARM) national user facility and the Atmospheric System Research (ASR) program. The research supports the Laboratory’s Global Security mission area and Science of Signatures capability pillar.
Reference: Gallo, F., Uin, J., Springston, S., Wang, J., Zheng, G., Kuang, C., Wood, R., Azevedo, E. B., McComiskey, A., Mei, F., Theisen, A., Kyrouac, J., and Aiken, A. C. “Identifying a regional aerosol baseline in the eastern North Atlantic using collocated measurements and a mathematical algorithm to mask high-submicron-number-concentration aerosol events.” Atmos. Chem. Phys., 20, 7553–7573; https://doi.org/10.5194/acp-20-7553-2020, 2020.
Technical contacts: Allison Aiken and Francesca Gallo
Materials Physics and Applications
From a Mott insulator to an ideal two-dimensional metal
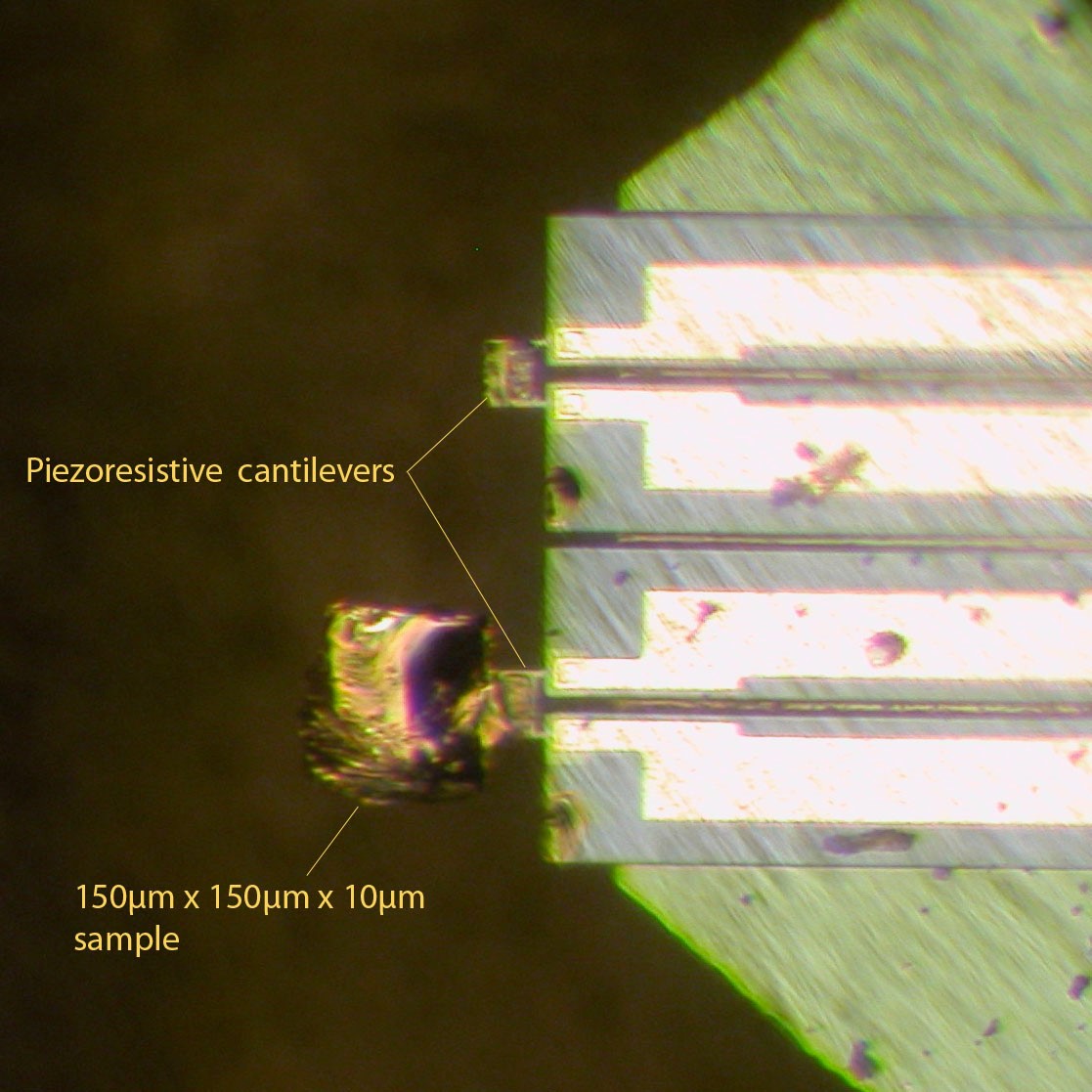
Sample on a piezoresistive cantilever
In high-temperature superconductivity, the pseudogap refers to a state of matter extending up to room temperature and beyond when layered copper oxides are doped with sufficient holes to transform a Mott insulator into a metal, but insufficient holes to achieve optimal superconductivity.
Because it spans both the Mott insulating and superconducting regimes, the pseudogap is believed to hold the key to understanding the origin of high-Tc pairing. However, considerable debate continues as to whether the pseudogap constitutes a novel form of order, and how exactly the gap manifests itself over the Fermi surface.
Recently, a team of researchers, including scientists from the National High Magnetic Field Laboratory-Pulsed Field Facility (MPA-MAGLAB), performed magnetic quantum oscillation experiments on the arguably highest-quality single crystals of the high-Tc superconductor YBa2Cu3O6+x (or YBCO for short) produced thus far and have made the surprising discovery that the material behaves much like an ideal two-dimensional metal.
Their work was published in the journal Nature Physics.
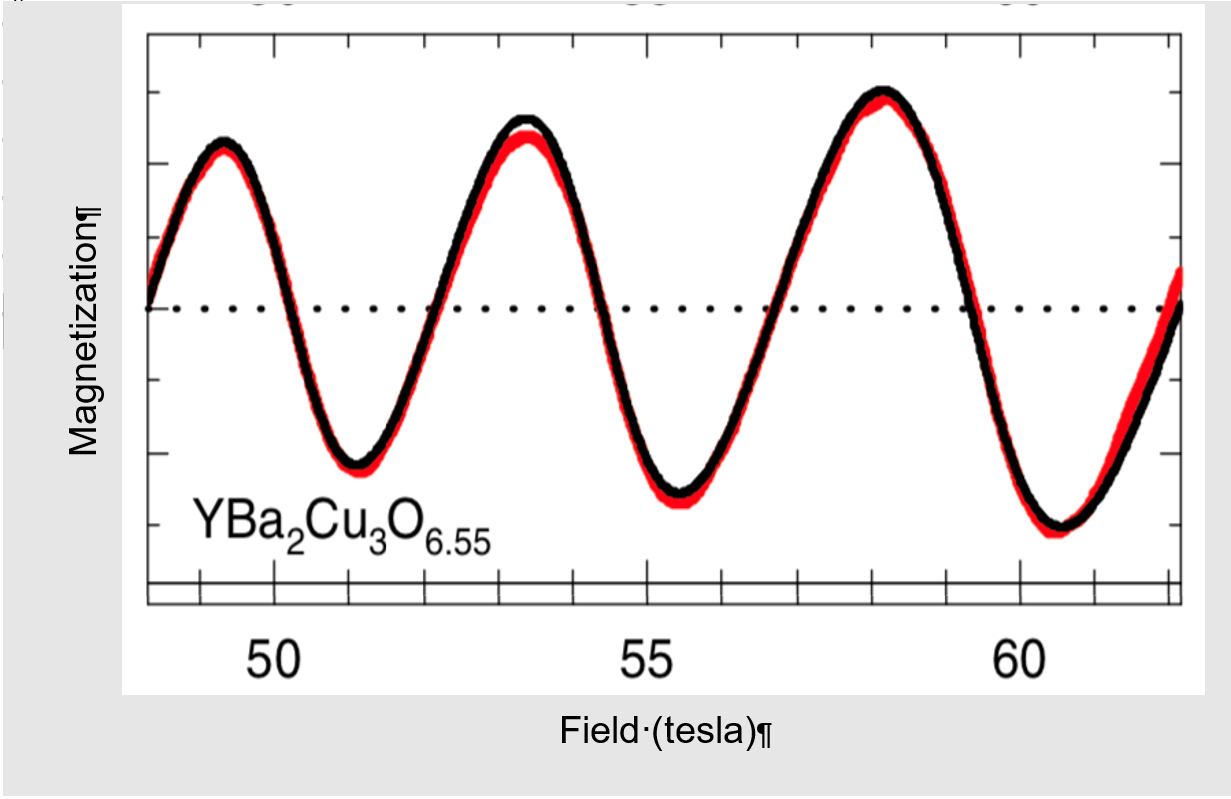
This graph shows an example of the distinctive “sawtooth” de Haas-van Alphen oscillations in a high-Tc superconductor
Ideal two-dimensional metals have known to exist in GaAs heterostructures for many years, but have recently also been show to occur in graphene and on the surface of topological insulators. What the quantum oscillation experiments show is that like GaAs, the Fermi surface consists of a single pocket of carriers that exists in complete thermodynamic isolation—it behaves as if the number of particles is rigidly fixed, causing the wave form of the de Haas-van Alphen magnetic oscillations to acquire a distinctive “sawtooth” wave form. Given that no carriers are exchanged with surroundings, the experiments imply that the pseudogap is what can be referred to as a hard gap. Thus, with the exception of the isolated pocket of carriers, the system is completely gapped very much like it continues to be an insulator.
The result is important because it significantly constrains possible origins for the pseudogap in high-Tc materials. One class of theories predicting the pseudogap to be heavily broadened by strong electronic correlations can essentially be eliminated. Rather, the existence of a hard gap with little or absent broadening points to a novel form of quantum or topological order within the pseudogap state.
The quantum oscillations were detected in the magnetic torque using a piezoelectric cantilever at the National High Magnetic Field Laboratory in strong magnetic fields extending to 65 tesla by a team of scientists including Neil Harrison, Mun Chan, Ross McDonald, and Kimberly Modic. The high-quality crystals were grown at the Max Planck Institute for Solid State Research in Germany, while the sample preparation was conducted at the Cavendish Laboratory in the United Kingdom. Additional supporting experiments were performed at the High Magnetic Field Laboratory in China.
Funding and mission
The research was funded by Basic Energy Sciences (Department of Energy, Office of Science) “Science of 100 Tesla” program. The work supports the Laboratory’s Energy Security mission area and falls under the purview of the Materials for the Future capability pillar.
Reference: Hartstein, M., Hsu, Y., Modic, K. A. et al. “Hard antinodal gap revealed by quantum oscillations in the pseudogap regime of underdoped high-Tc superconductors,” Nature Physics, 4 (2020). https://doi.org/10.1038/s41567-020-0910-0
Technical contact: Neil HarrisonMaterials Science and Technology
Rare experiment leads to discovery of new material defect mechanism
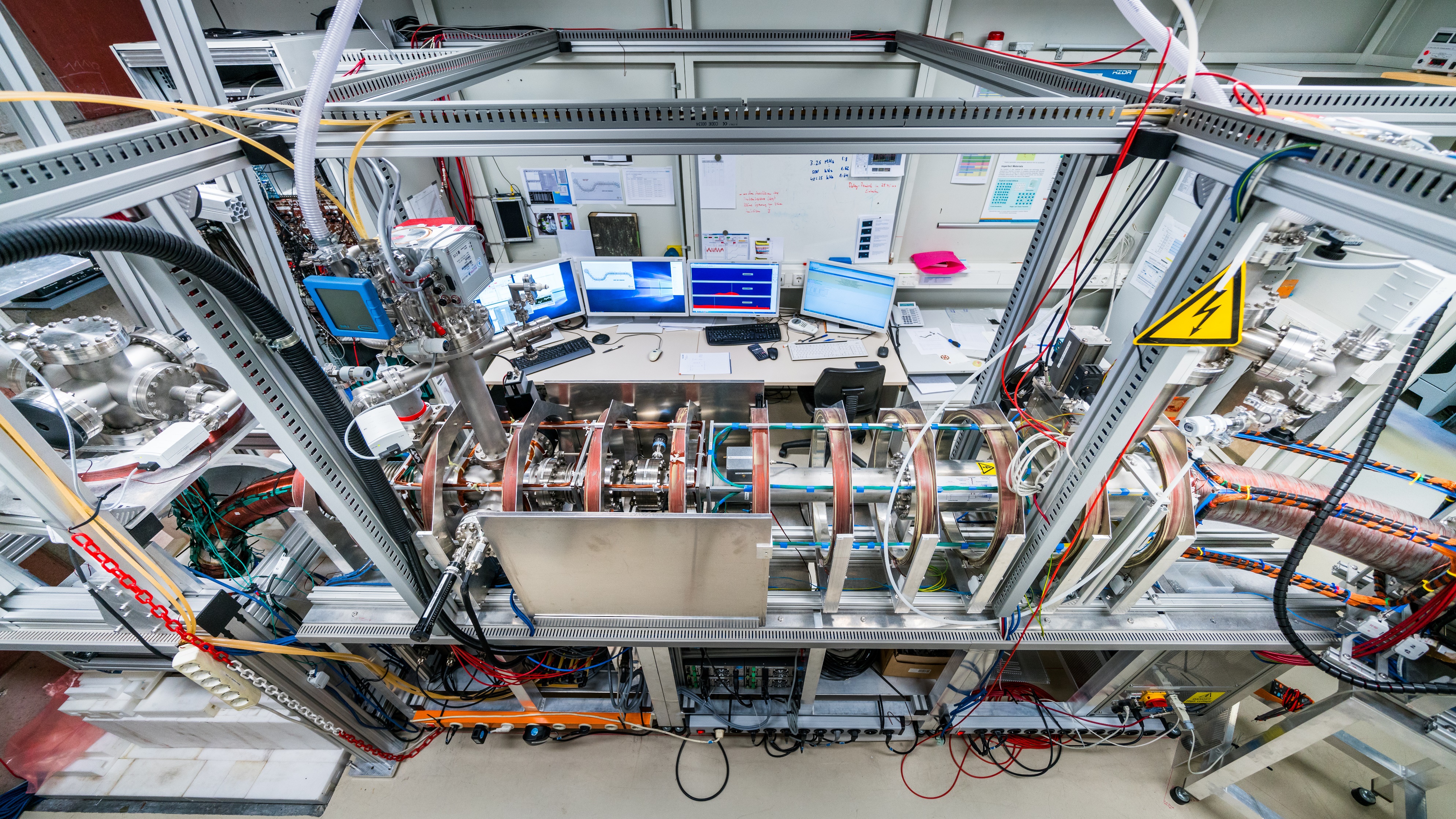
Experimental setup of the positron system at Helmholtz-Zentrum Dresden-Rossendorf in Germany (photo credit: HZDR/A. Wirsig).
A new material defect mechanism was discovered after Los Alamos researchers and their collaborators achieved a technically challenging experimental setup that employs a rare capability.
As part of Fundamental Understanding of Transport Under Reactor Extremes (FUTURE)—an Energy Frontier Research Center led by LANL—researchers in Materials Science and Technology (MST) and Materials Physics and Applications (MPA-CINT) conducted this unique experiment with collaborators at Bowling Green State University, Helmholtz-Zentrum Dresden-Rossendorf, North Carolina State University, the University of California Berkeley, and Pacific Northwest National Laboratory. Their results were published in Science Advances.
The experiment took advantage of the newly developed short-pulsed positron beam in Dresden, Germany. This was the first experimental study to use a nondestructive depth-resolved positron annihilation lifetime spectroscopy (PALS) beam to probe the damage profile in ion-irradiated metallic systems.
Radiation damage, which causes material defects, is a research topic of high importance, and this experiment contributes data needed to improve material defect simulations.
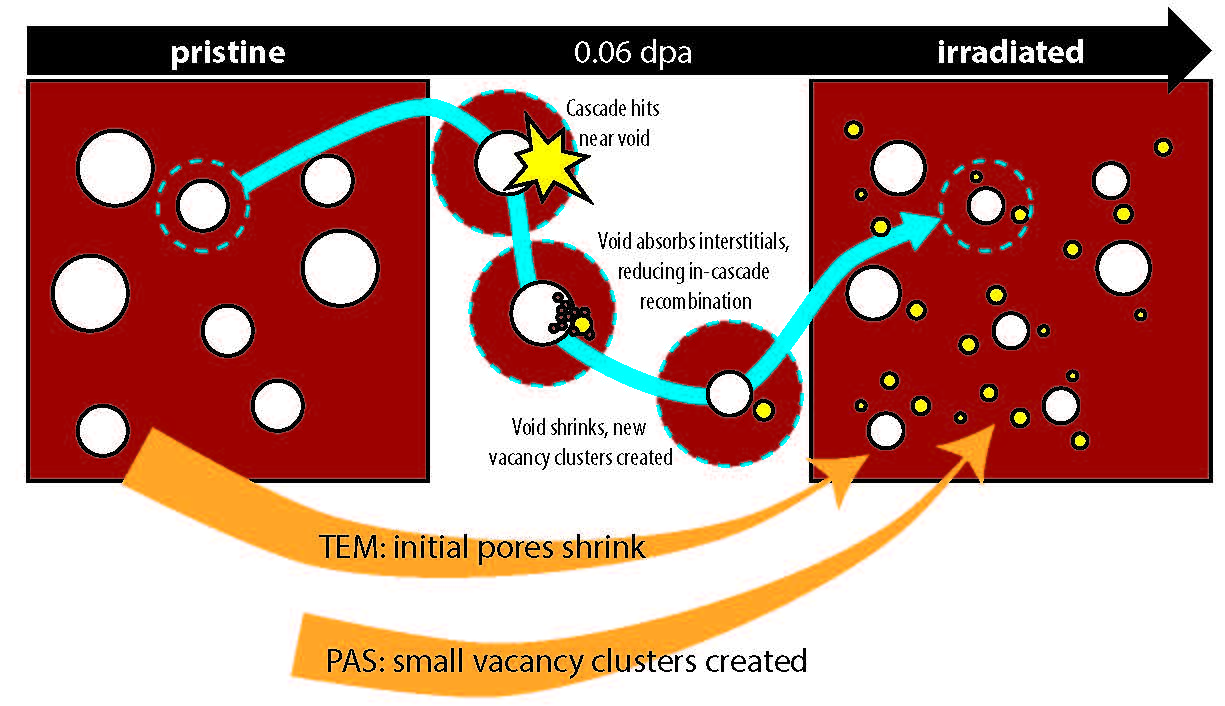
The new mechanism revealed by this study highlights the competing effects of sinks in the material.
Understanding materials in extreme environments
An ongoing field of study consists of predicting and understanding how materials will behave in extreme environments, such as radiation conditions. Radiation can cause a variety of material defects, such as dislocation loops, vacancies, and voids, which lead to altered material properties: swelling, hardening, and embrittlement.
Scientists and engineers want to know that their materials will be able to withstand these extreme environments, without catastrophic failure. However, experimental capabilities for probing defects under irradiation have their limitations, particularly when it comes to the very smallest defects that are created.
Nondestructive and depth-resolved
The researchers combined depth-resolved Doppler broadening and PALS with transmission electron microscopy to identify vacancy clusters in ion-irradiated iron and measure their density as a function of depth. This combination of experimental techniques led to the discovery of an unknown mechanism — one that details the interaction of cascade damage with voids in ion-irradiated materials. The mechanism explains the observation that cavities actually shrink when irradiation occurs.
The mechanism is described as this: the irradiation creates a cascade of interstitials that flow preferentially to the existing voids in the material, which act as a sink. This causes the voids to shrink. However, because the interstitials are sucked away by the voids, the vacancies that are also created by the cascades accumulate faster than normal. This mechanism highlights the competing effects of sinks in the material.
Funding and mission
This work was supported as part of FUTURE (Fundamental Understanding of Transport Under Reactor Extremes), an Energy Frontier Research Center funded by the U.S. Department of Energy, Office of Science, Basic Energy Sciences. This work was performed, in part, at the Centers for Integrated Nanotechnologies (CINT), an Office of Science User Facility operated for the U.S. Department of Energy Office of Science.
The work supports the Laboratory’s Energy Security mission and the Science of Signatures capability pillar.
Reference: S. Agarwal, M. O. Liedke, A. C. L. Jones, E. Reed, A. A. Kohnert, B. P. Uberuaga, Y. Q. Wang, J. Cooper, D. Kaoumi, N. Li, R. Auguste, P. Hosemann, L. Capolungo, D. J. Edwards, M. Butterling, E. Hirschmann, A. Wagner, and F. A. Selim. “A new mechanism for void-cascade interaction from nondestructive depth-resolved atomic-scale measurements of ion irradiation–induced defects in Fe.” Science Advances. Vol. 6, No. 31, 2020. https://doi.org/10.1126/sciadv.aba8437
Technical contact: Blas Uberuaga
Foundational research for emerging class of materials: oxysulfide perovskites
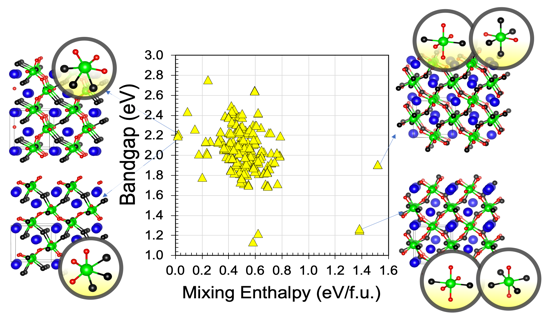
Anion-order-dependent variation in energetics and bandgaps for CaHf(O0.5S0.5)3. The panels show some of the most favored and unstable local anion configurations.
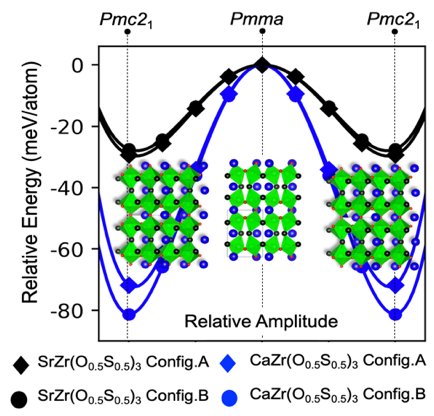
Computed ferroelectric well-depth for a selected set of configurations for the two most stable ferroelectric oxysulfide chemistries identified in this study.
Heteroanionic oxysulfide perovskite compounds represent an emerging class of new materials that allow for a wide range of tunability in electronic structures, making them promising for use in novel and diverse applications, including solar energy harvesting, catalysis, and sensing.
In work published in NPJ Computational Materials, Los Alamos researchers, in collaboration with researchers from the University of Connecticut and Washington University in St. Louis, performed a combined first-principles-based electronic structure and machine-learning analysis for a prototypical class of anion-ordered oxysulfide perovskites. Their results develop a holistic understanding of various factors that dictate anion ordering tendencies in these compounds and, more importantly, reveal implications on stability and functionality for this class of materials.
Until now, anion ordering in heteroanionic perovskites, such as oxysulfide perovskites, was poorly understood. The presence of two anions influences the structure and properties of the compound.
Leveraging the Laboratory’s expertise in computer modeling and machine learning, the researchers used density functional theory computations and materials-informatics techniques to show that the material’s ordering tendency is a combined stabilization effect stemming from electronic, elastic, and electrostatic contributions, regardless of specific cation chemistries.
“For instance, as a general rule we found that a cis-arrangement of O and S around the BX6 octahedra is highly preferred over a trans-arrangement, irrespective of the A- and B-site chemistries,” said Ghanshyam Pilania of Materials Science in Radiation & Dynamics Extremes (MST-8).
Additionally, the researchers found that the bandgap is controlled by crystal field splitting imposed by the surrounding anion in the BX6 octahedron, and the polarity induced as a result of the cis-arrangement can potentially support ferroelectricity. These fundamental findings are a vital step in the endeavor to design these materials that offer intriguing possibilities for novel functionality.
Funding and missionThe Los Alamos portion of the work was funded by the Laboratory Directed Research and Development (LDRD) program. Computational support for this work was provided by LANL’s high-performance computing clusters. The work supports the Laboratory’s Energy Security mission area and the Materials for the Future and Information Science and Technology capability pillars.
Reference: “Origins and implications of anion order in oxysulfide perovskites.” NPJ Computational Materials. 6, 71 (2020). https://doi.org/10.1038/s41524-020-0338-1
Researchers: Ghanshyam Pilania, Steven Hartman, Christopher R. Stanek, Blas P. Uberuaga (Materials Science in Radiation and Dynamics Extremes, MST-8); Rohan Mishra (Washington University in St. Louis); and Ayana Ghosh (University of Connecticut).
Technical contact: Ghanshyam Pilania
Physics
Los Alamos scientists author key chapters for Deep Underground Neutrino Experiment design
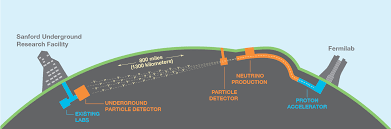
The Deep Underground Neutrino Experiment comprises a neutrino beam with a near detector located at Fermi National Accelerator Laboratory in Illinois and a far detector located 800 miles away in South Dakota.
The Deep Underground Neutrino Experiment (DUNE) is an international, world-class experiment aimed at studying peculiar particles called neutrinos. From these neutrinos, scientists will better understand the matter–antimatter imbalance in the universe. In fall 2020, key technical design reports for DUNE were published, and Los Alamos scientists authored and edited several of the chapters.
“The Technical Design Reports encompass an enormous body of work, including detailed technical designs and prototyping work performed in the last two years along with physics,” said Sowjanya Gollapinni of Subatomic Physics (P-25).
Three of the five DUNE volumes were published in a special issue of the Journal of Instrumentation. The completion of the technical design was momentous, and construction will soon commence, with a goal to collect first physics data in the late 2020s.
Introduction to DUNE
Volume one serves as an executive summary of the DUNE science program. It provides detail into what drove the choice for the detector technologies selected.
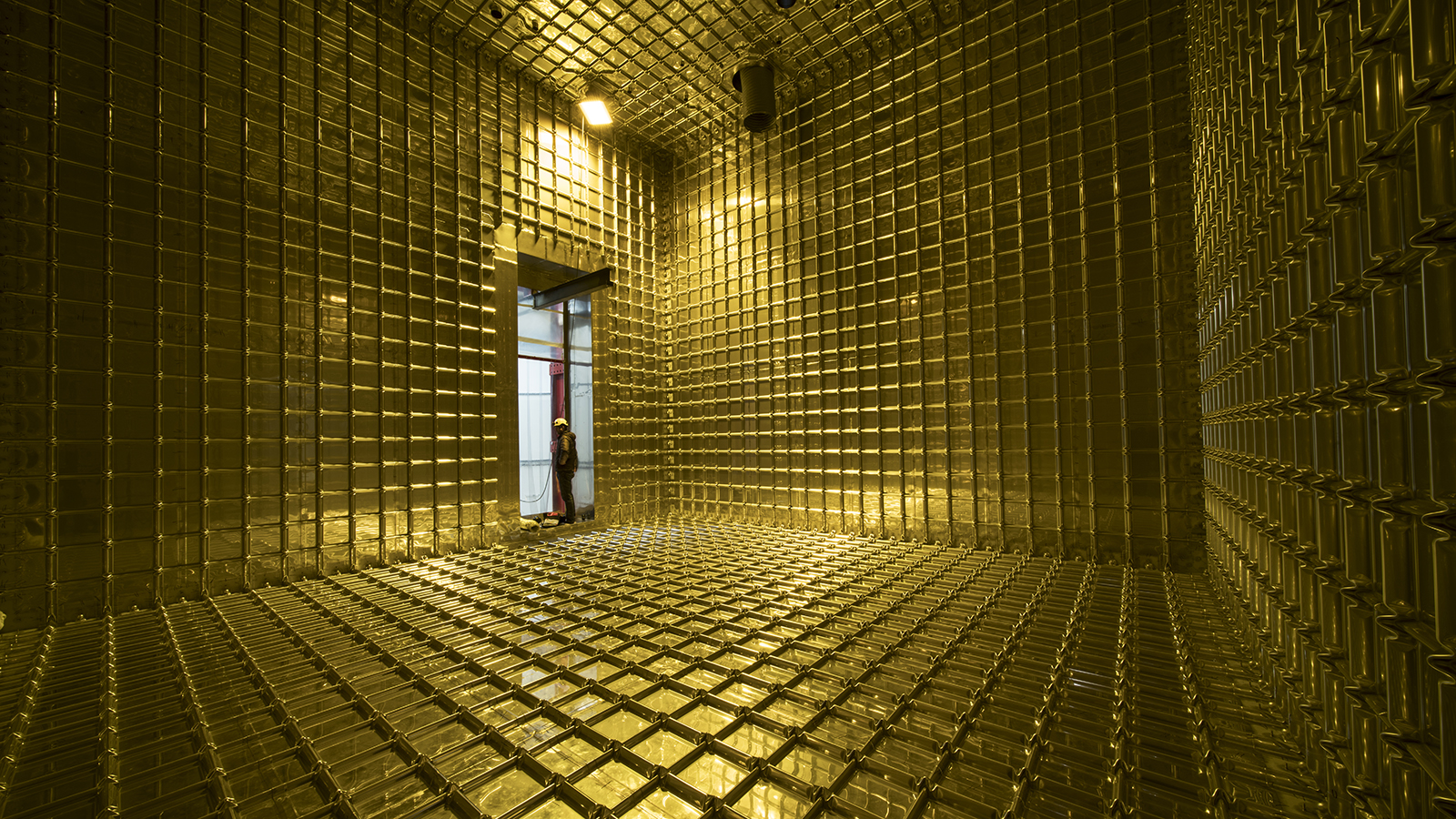
The ProtoDUNE single-phase experiment is under construction at CERN in Geneva, Switzerland.
Neutrinos exist in three flavors (electron, muon, and tau) and have the ability to change from one flavor to another, a phenomenon referred to as neutrino oscillations. By comparing how neutrinos and their counterparts (anti-neutrinos) oscillate, DUNE can offer insight into the mystery of matter–antimatter asymmetry.
The experiment is comprised of a near detector located at Fermi National Accelerator Laboratory in Illinois and a far detector located 800 miles away in South Dakota.
Far detector technical coordination
Volume three focuses on the far detector, which is housed 1.5 km underground at the Sanford Underground Research Facility in Lead, South Dakota. A consortium of entities, one of which was Los Alamos, determined the design and construction of this massive detector.
The far detector is capable of holding 40,000 tons of frigid liquid argon and equipped to detect thousands of neutrinos from a supernova far, far away. In particular, DUNE is sensitive to the electron-neutrino flavor and its oscillations after propagating a long distance in earth (called matter oscillations). This is a unique capability.
Far detector single-phase technology
Volume four details one of the two prototyped options for the far detector: the single-phase liquid argon time projection chamber (LArTPC). Single phase offers a highly modular design with four drift volumes, each with 3.5 m of horizontal drift, whereas the other prototype (the dual phase) offers 12 m of vertical drift.
The DUNE far detector consists of four distinct detector modules, thus allowing for flexibility in design. The first two far detector modules will be single-phase LArTPCs. The third module is expected to be a dual phase, and the technology for the fourth module is undecided—opening the door for exploring novel detector technologies.
Los Alamos led the calibration, cryogenic instrumentation, and slow controls efforts for the DUNE Technical Design Report as well as authoring related chapters. These volumes represent a significant milestone for DUNE.
DUNE’s timeline
Officially, ground was broken for the DUNE project in July 2017. The far detector main cavern excavation is actively ongoing. The first far detector is anticipated to start installation in 2024, with a goal to collect first physics data in the late 2020s.
DUNE prototype detectors, ProtoDUNEs, which are an essential step in testing new technologies for DUNE at a large scale, have been online since 2018 at CERN in Geneva, Switzerland. These prototype detectors are taking data and offering insight into how detector scale-up for DUNE could work; the prototypes also provide valuable validation of the planned design for DUNE.
Funding and mission
The reports were prepared by the DUNE collaboration using resources of the Fermi National Accelerator Laboratory (Fermilab), a U.S. Department of Energy, Office of Science, HEP User Facility. The research supports LANL’s Global Security mission area and the Science of Signatures capability pillar.
References:
DUNE Far Detector Technical Design Reports. JINST. Vol. 15. 2020.
Vol I: https://iopscience.iop.org/article/10.1088/1748-0221/15/08/T08008
DUNE Physics Volume: https://arxiv.org/abs/2002.03005.
Technical contact: Sowjanya GollapinniICF advancement: First observation of scale invariance in the high-energy-density regime
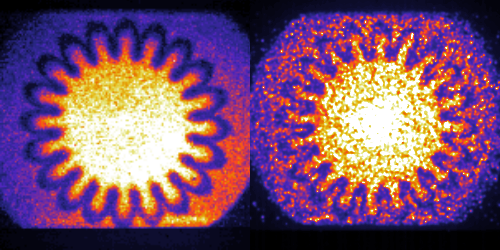
X-ray radiograph of direct-drive cylindrical implosion at the Omega Laser Facility (left) and the National Ignition Facility (right). The cylinders have an initial mode = 20 engineered sine-wave perturbations. The perturbations grow largely due to Rayleigh–Taylor instability as the cylinder implodes.
Los Alamos inertial confinement fusion (ICF) researchers along with colleagues demonstrated scale-invariant hydrodynamic growth for the first time. Using two different ICF facilities—the National Ignition Facility (NIF) at Lawrence Livermore National Laboratory and the Omega Laser Facility at the University of Rochester—the researchers measured the same hydrodynamics over spatial and temporal scales that vary by a factor of three.
“Precise measurements of hydrodynamic instability growth are crucial for validating the underlying physics models in radiation-hydrodynamics codes central to ICF experiments as well as improving predictive capability,” said Sasi Palaniyappan of Plasma Physics (P-24).
The benefits of different scales
The National Ignition Facility is larger than the Omega facility, and there are important benefits attributed to this difference. For one, a smaller facility means more experiments (or “shots”) can be performed in a given day — approximately 15 shots per day at Omega versus one shot per day at NIF. The cost of resources at Omega is also lower.
Therefore, it is quite valuable to know that these smaller experiments at Omega can accurately represent the larger experiments performed at NIF. The researchers demonstrated this through implosions of cylindrical targets by measuring instability growth in the deceleration phase.
The NIF cylinder target was three times larger in radial dimension compared to the Omega cylinder, which was roughly 1 mm in diameter. The cylinder housed an embedded aluminum marker layer with precisely engineered perturbations (mode = 20), enabling instability growth measurement via X-ray radiography. The NIF implosion also took three times longer compared to the Omega implosion.
Instabilities cause performance degradation
Hydrodynamic instabilities must be understood and overcome in order to achieve burning plasma. In particular, two hydrodynamic instabilities, Rayleigh–Taylor and Richtmyer–Meshkov, cause significant performance degradation in ICF implosions. These instabilities lead to fuel-pusher mix, reduced hot-spot temperature, reduced compression, and lower neutron yield.
Unfortunately, these instabilities are common and can be seeded by imperfections, such as surface roughness, drive non-uniformity, and engineering defects: fill tubes, glue spots, and tents. Once seeded, the instabilities grow, leading to mix and performance degradation of ICF implosions. Understanding such instability growth and ways to mitigate it are vital to achieving ignition in ICF capsules.
The researchers measured single-mode (m = 20) instability growth factors at a convergence ratio (i.e., final-to-initial radius of the fuel core) of approximately 2.5. They observed nearly identical mode growth at both scales, and the measurements were in agreement with xRAGE radiation-hydrodynamics simulations. In the process, they developed a Bayesian-inference-engine method to account for the variations in the target alignment, magnification, and the parallax effect in the measurement, allowing a more precise comparison between the experimental data and the simulations.
The researchers plan to increase the implosion convergence ratio from the current 2.5 ratio to approximately 10–15 by replacing the foam core with low-density gas fill. Measurements at high convergence will provide instability measurements directly relevant to ignition-scale implosions.
Funding and mission
The work, which supports the Laboratory’s Nuclear Deterrence mission area and the Nuclear and Particle Futures capability pillar, is funded by the Inertial Confinement Fusion program (LANL Science Campaign 10, Program Manager John Kline).
Researchers include Sasi Palaniyappan (Plasma Physics, P-24), Joshua Sauppe (Plasma Theory and Applications, XCP-6), Kirk Flippo (P-24), Benjamin Tobias (Neutron Science and Technology, P-23), and John Kline (Office of Experimental Sciences, OES).
References:
- P. Sauppe, et al. “Demonstration of scale-invariant Rayleigh-Taylor instability growth in laser-driven cylindrical implosion experiments,” Phys. Rev. Lett. 124, 185003 (2020). https://journals.aps.org/prl/abstract/10.1103/PhysRevLett.124.185003
- Palaniyappan, et al. “Hydro-scaling of direct-drive cylindrical implosions at the OMEGA and the National Ignition Facility,” Physics of Plasmas 27 (4), 042708, (2020). https://doi.org/10.1063/1.5144608
- P. Sauppe, et al. “Design of cylindrical implosion experiments to demonstrate scale-invariant Rayleigh-Taylor instability growth,” High Energy Density Physics, 36, 100831 (2020). https://doi.org/10.1016/j.hedp.2020.100831